The
Physiology of Transport Substances in the Blood (Sodium)
By Professor Marcel Uluitu, M.D. Ph.D.
Spiru Haret University
Bucharest, Romania
Co-Authored by Diana Popa (Uluitu), M.D.
Department of Microbiology, Immunology
and Molecular Genetics
University of Kentucky, Lexington,
Kentucky, USA
[Editor’s Note: This paper is presented as Part II of a
series of chapters from the new book “The Physiology of Transport Substances in
the Blood (Sodium)”; subsequent chapters will be featured in upcoming issues of
this Journal. This segment features Section II (of three sections) of Chapter
Two (of six chapters)]
MOTTO:
Measure what is measurable
and make measurable what is not,
-- Galileo Galilei (from Ev.Z. 2008)
2.5.7.4. Aminoacid composition
Plasma proteins contain all the 20 amino acids, but in different quantities.
Best represented acids are: aspartic acid, glutamic acid(139), leucine and least represented are tryptophan,
glycine, etc.
Table no. 7 The
composition of amino acids in the plasma protein (gr/100gr of protein) (139)
lisine
12, 3
5, 03
10, 62 9,
15 8, 01
histidine
3, 5
1, 31 2, 61 3, 12 2, 55
arginine
6, 15
3, 65
6, 97 5,
03 4, 45
aspartic acid
10, 4
7, 44
8, 26 11,
37 9, 05
threonine
5, 0
4, 80
5, 64 3, 75 8, 90
serine 3, 7 2, 51 5, 64 4, 28 11 75
glutamic acid
17, 4 10, 73 19, 20 9, 46 12, 49
Proline
5, 1
2, 37
4, 12
4, 20 7, 90
Glicine
1, 6
0, 82
2, 53
3, 98 4, 47
Alanine - - 5, 63 5, 47 4, 05
Cysteine
0, 70
0, 60
3, 15
- -
Valin 7, 70 2, 82 6, 41 5, 05 9, 42
Methionin
1, 28
0, 65
- 1, 53 0, 90
Isoleucin
1, 70
3, 85
-
2, 02 2, 59
Leucin
11, 90 5, 21 17, 67 8, 21 8, 57
Tirosin
4, 06
1, 99
3, 43
4, 91 6, 75
Phenylalanine 7, 80 3, 02 4, 69 4, 90 4, 79
Tryptophan
0, 19
1, 25 - 1, 66 3, 42
Plasma
protein metabolism. Turnover of proteins is variable, according to the fraction
considered. Globulins have a slower turnover. Protein synthesis is stimulated
by some intermediates (130, 139). Degradation products of prothrombin
stimulate its own synthesis. Cytokines have different effects on the
well-differentiated hepatocytes with effects on acid
phase protein synthesis and on plasminogen, etc.
2.3.5.8. The main plasma proteins.
2.3.5.8.1. Prealbumin.
It is an albumin rich in tryptophan. Electrophoretically, it has more mobility than albumins. It
contains sugars (hexose, hexozamine)
GM = 61,000. Plasma content is 30% mgr. Its most important role is that of thyroxine transporter.
2.3.5.8.2
.Albumin.
Albumin is the
most significant fraction in point of quantity. Together with prealbumins, it migrates the fastest on the electrophoregram.
Plasma content is 4.5-5.5 gm%. It is very soluble in water. It has a small
molecule with G.M. = 69,000. It has an important role in colloid-osmosis,
adjusting the hydro electrolytic equilibrium, in blood transport processes. Its
molecule is spherical or ellipsoidal. It is a polydomenial
molecule and has only amino acid residues (Table No. 7). The electrical charge
is given by 96 lateral negative groups and a similar number of positive ones.
At the physiological pH of the blood - 7.4, it has a large number of negative
charges, 12 -15 COOH dissociated protons, histidil
which gives more stability. The molecule is surrounded by a layer of water. Electrophoretic mobility in veronal
buffer is 6.7 -7.4 x 10
/cm
/V/sec. The isoelectric
point is pH = 4.7. The refoldings are fixed by disulphur bridges, which favor the binding of heavy metals.
Albumins are resistant to denaturizing, which is important in the plasma. It is
synthesized in the liver. Albumin is distributed between blood and the extra
vascular space, having a small molecule. Half of its value in blood helps to
maintain volume equilibrium between the two compartments and in transport
processes. Synthesis depends on their intake of amino acids, on some hormonal
actions (thyroxine, steroid hormones). Synthesis is
stimulated by the loss of protein, by the products of their degradation and by
the action of cytokines. They provide 75 - 80% of the colloid-osmotic pressure
of plasma. They have also a role in maintaining the acid base equilibrium,
reservoir of amino acids. They also bind carbon hydrates, hydrophobic compounds
as free fatty acids, liposoluble vitamins, steroid hormones. For binding, a pocket of hydrophobic amino
acid is formed that has a collar of cationic hydrophilic amino acids. Fatty
acids are attached with their lyophilic end to the
pocket and with their hydrophilic end to the collar (COO). More than half of serum lipids are transported on albumin even chilom. Serine protease inhibitor (serpine)
is a group of glycoprotein in serum with inhibitory action on protease having
protective action on the body. They represent about 10% of the total protein
levels. The group includes antitrypsin, antichimotrypsin, antithrombin
III, protein C inhibitor, macroglobulin. Inhibitory activity is relatively
specific. They have no role in the transport of substances through blood.It is synthesized in the liver, macrophages,
endothelial. GM = 735,000.
icrons. It binds the biliary
salts, acids coloring, drugs (atropine, quinine, pilocarpine,
digitalis, etc.). In pathology, hyperalbuminemia is
insignificant. Hypoalbuminemia accompanies the nephrotic syndrome, chronic hepatitis, acute and chronic
digestive diseases, burns, bleeding, malnutrition, etc.
2.3.5.8.3. Glycoproteins.
They are compounds that contain carbohydrates covalently bound by proteins.
Almost all glycoproteins contain hexozamine
(glucozamin, galactozamin)
and monosaccharides. Sialic
acid is a frequent component (Table No. 8). It confers molecules some physical
and chemical properties. The saccharoid fraction has more frequently a branched structure and consist
in saccharoid residues. Sialic
acid indicates the constant presence of glycoproteins.
They are present in all globulins.
Table. 8.The% content of sugars in proteins (139).
---------------------------------------------------------------------------------------------------------------------------------------
Protein Hexoze Hexozamin Sialic acid
Fucose
---------------------------------------------------------------------------------------------------------------------------------------
prealbumin 1, 1 0, 15 0 0

glycoprotein 15, 0 12, 00 12, 00 1
ceruloplasmin 3, 0 1, 90 2, 00 0, 18
haptoglobin 7, 8 5, 30 5, 20 0, 20

macroglobulin
3, 6 2,
30 1, 80 0, 12
Acid 
glycoprotein
12, 0 13, 00 17, 00 0, 60
transferrin 2, 4 1, 60 1, 40 0, 07
fibrinogen 3, 2 1, 00 0, 80 0, 00

glycoprotein 6, 7 5, 80 4, 40 0, 20
2.4. Inorganic compounds of plasma.
2.4.1. Water.
It is a major constituent of living things and of the environment. It
represents, on average, 80% of the living body with relatively large variations
from one tissue to another. Water is "le milieu interieur"
according to Claude Bernard (1878). In the absence of water is cells go
dry. Depending on their position on the
evolutionary scale, or on special conditions they die or can revive their
activity in the presence of water (68). Water has special properties compared
to other substances, which determines the Nature of the physical and the
biological world. It is part of the cell structure. It is the environment in
which biochemical processes and physiochemical processes are carried out. In
higher living things, water is distributed in the three spaces-Intravascular
(5%) interstitial (15 -30%) and intracellular (40 - 50%) or, in average figures
(44) in a body of 70 kg, water represents approximately 50 liters, of which 35
l is intracellular water, 3.5 l blood plasma, and 11,5 l interstitial water.
(43).
2.4.1.1. The structure of water
The structure of water (68) enables the understanding
of its remarkable properties. A molecule of water has the shape of an isosceles
triangle, a dipole arising from the existence of the two covalent links of the
two atoms of hydrogen with oxygen (length = 0.99 A) which form an angle of 104
,58, sides O ---- H being equal. Covalent links separate the
negative charge of the oxygen atom from the positive charges of the atom of
hydrogen. A dipole is formed with intensive electronic attraction to oxygen
which turns negative the local area and leaves the hydrogen area with net
positive charge. Since only two electrons in the layer 8 of oxygen interact
with 2 protons, other two electrons attract two other charges of positive
hydrogen from another molecule. Thus, a
group of water molecules is formed having the shape of a tetrahedron around the
atom of oxygen, leaving the positive charges of a molecule of water to move to
the other negatively charged area of another neighboring molecule. Every
molecule of water thus tends to have four neighboring molecules, and each oxygen
atom is the center of a tetrahedron with other oxygen atoms at a distance of
2.76 A (68). This structure is encountered not only the ice but is maintained
up to the temperature of steam as a quasicrystaline
structure. (Fig-3)
Figure 3. Water structure (68)
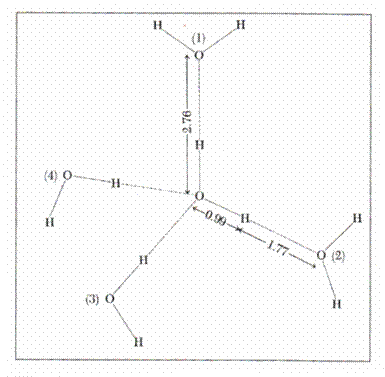
2.4.1.2. The properties of water.
Water is a transparent, clear liquid, colorless in thin layers (53) It is a good solvent. It has a very high dielectric constant
(80 to 20
C) depending on the dipole momentum.This
explains its capacity of good solvent and of an environment of electrolytic
dissociation. The density of water is highest at 4
C and decreases in both directions of temperature,
explaining why ice volume increases compared to water and why ice floats. Its
freezing point is 0
C and its vaporization point is 100
C.
Other physical properties of importance in biology are specific heat (the
amount of heat absorbed by the body - water - to raise its temperature by 1
C) and latent heat (the amount of heat required to change the
physical state of a body - water) both playing a particular role in
thermoregulation. These unusual physical properties of water are due to the
intense intermolecular attraction through the hydrogen bonds located between
the covalent bond and the weaker Van der Waals forces. This type of interaction is electrostatic and
binds two electronegative atoms (N, O)
2.4.1.3. Water in the body.
Water is the environment in which vital processes are carried out: biochemical,
physical-chemical and physiological processes, by means of the properties
listed. It acts as a solvent in the processes of absorption, excretion and in
the transportation of dissolved substances and of gas among the three
compartments in the processes of thermoregulation and osmoregulation,
and as an organic and inorganic solvent. The electrostatic interactions between
the water dipole and some dissolved substances lie at the basis of ionic
hydration as crystallization water. Aggregates formed by molecular hydrogen
bonds in the form of cavities, as hydrates, identified as "clatrate”
compounds have a still insufficiently known role in biology.
2.4.2. Sodium.
2.4.2.1. Chemistry of sodium
It is an alkaline metal in the same group as Li
, K
, Cs
, Rb
(153). It is very widespread in Nature in the form of salts
(nitrates, carbonates, chlorides). NaCl is a crystallized
substance containing aggregates of cations (Na
) and anions (CL
), each of them surrounded by six atoms with opposite charges
that are very hydrophilic. It is soluble in water. In an electric field, Na+ migrates to the cathode (Faraday, 1854).
Sodium ions are unpolarized. It is surrounded by six
molecules of water with a large diameter when in a hydrated state (Tb, 9). It
has one electric orbital less and it interacts with the water dipole.
Table 9: Influence of hydration on the dimensions of ions (51).
ion in
crystal
hydrated
Na
0,
95
3, 58
K
1,
33 3, 31
Cl
1,
81
3, 32
Br
1, 95 3,
30
I
2,
16
3, 79
2.4.2.2. Distribution of sodium.
It is present in both reigns. In the animal body, sodium is present in the
three compartments (43, 44), in varying, specific concentrations. It is present
in blood and in other liquid media (53) of the body, the interstice, including
in the form of deposits, and in the endocellular
medium.
2.4.2.3. Equilibrium of sodium in the three spaces of
the body.
Its distribution in the three spaces and among the
components of each space complies with the laws of physical and chemical
equilibrium (3, 57) with different functional meanings as follows: Equilibrium
between intravascular Na (44) and extra vascular (interstitial) Na and between
that the latter and intracellular Na. Within the intravascular space, there is
a balance between Na in interaction with blood proteins and chemically active
Na. In the extra vascular space (51) with a heterogeneous composition, there is
equilibrium of sodium, between the forms of deposit, with Na in the liquid
matrix. Na balance between the three spaces through separating membranes is
achieved by diffusion (85) together with other substances such as water, K,
etc. amino acids, and by active processes with various speeds. Water becomes
uniform in the blood and the interstice within 30 seconds while the balancing of
Na requires 60 minutes. Interstitial balance and intracellular balance is
obtained for radioactive water in 120 minutes, for Na
in 24 hours, and for in K
in 25 minutes, showing that Na adjusting is independent of
that of water (43). These types of general equilibrium are dependent on the
balance between intake and elimination of the cation
described by a polynomial (222, 223. 227). The total amount of Na in the body
is 3500 - 4500 mEq of sodium (80 - 100 gm) (43, 44).
It is present in an osmotic inactive form, of which 500 mEq
of sodium (11.5 gm) in connective tissue, cartilage and 1.400 - 1900 mEq of sodium (32 - 45 gm) in the bones, skin tissue and
adipose tissue. 30% of Na is osmotically active and
participates in the tensio-osmo-regulation process.
Of the total Na, 2800 to 3000 mEq of sodium, (41 -42
gm / kg) is the amount of exchangeable cation (Ganong and Harper cited by 44). This shows the active
exchange between fixed, osmotically inactive Na (35,
57, 56) and Na circulating in the interstitial fluids
and blood. This distribution of Na shows that in addition to metabolic, (34)
osmotic and rheologic factors, different physical and
chemical factors also have a contribution such as the multitude of anionic
groups interacting with Na (240)
2.4.2.4. Intravascular Na.
It circulates in the blood under two forms: bound and in an ionic, chemically
active state. The summation of the two forms represents total natremia. Its value (145 mEq ‰ of
sodium is a biological constant with close limits of variation: hyponatremia (136 mEq of sodium)
and hypernatremia (160 mEq
of sodium) (8, 187). Exceeding of these limitations (74) is associated with
general disturbances which, uncorrected, are incompatible with life.
|
2.4.2.5. Blood homeostasis of Na.
Blood Na homeostasis is maintained by complex neurohumoral
and physical-chemical, biological mechanisms. In the capillary membrane, Na
in excess goes into the interstice and is stored in the connective tissue,
bone, and subcutaneous fat (82). In hyponatremia,
correction is achieved by mobilizing deposits of Na, the exchangeable
fraction. Other mechanisms recognize changes in blood mass through the
migration of water in the tissues hypernatremia and
dilution of blood Na, or its passage into tissues, that become inundated, in hyponatremia. In pathological conditions, there may
develop an imbalance between the Na content of deposits and its plasma
concentration. The resulting hypernatremia draws
attention to the fact that the possibility of fixing Na on the deposit sites
have been exceeded, or to the loss of water in relation to the content of Na
(7, 75), when along with increased protein concentration there is increased natremia. Hyponatremia occurs
(8) by Na loss: sweating, diabetes mellitus, gastrointestinal disorders,
burns, hypodipsia, impaired adrenal cortex (14,
212) or after administration of drugs (67), lithium, vasopressin, etc.
|
2.4.2.6. The role of sodium.
It is a cell activator (61). It plays a decisive role in the processes of cell
excitability (83), the genesis and transmission of action potential (28). It
influences the accumulation of amino acids in the extra cellular space.Na acts as an enzymatic activator (152). It induces allosteric changes to thrombin in blood coagulation (9,
40.49). Sodium is involved in the acetylcholinergic
synaptic transmission (4) since it can pass through the channels of
acetylcholine (94, 106). In the interstitial space, it forms electropositive, pericellular clouds with a role in the genesis of action
potential (174, 179, 4). In baby rats (2 - 21 days
old) there is a high density of Na currents around the cortical neurons that
appears to control their intrinsic excitability at that age (6)
2.4.2.7. Determination of plasma sodium. (2, 3, 43, 59, 58, 131, 101, 153, 211)
The interest for sodium has stimulated elaboration of a great number of methods
for its quantitative determination in biological fluids and in the excitable
structures. It occupies a central position in mental activity (179). Dosing it
is difficult. It is diamagnetic. Compounds in which it is included have little
coloring. To this, one should add the heterogeneity of the biological
environment: high content of electrolytes, amphoteric
substances, macromolecules, etc. Moreover, measuring of total Na content is
irrelevant for deciphering its physiological role. For this purpose it is
necessary to determine the active chemical Na, including the balance between
the bound forms and the free, active form, membrane medium included (29, 35,
68, 149). Therefore, one is interested in the total content of Na, the
concentration of chemically active Na the strength of binding forms
interactions and the Nature of the transporter. Dosing methods are well-known
biological, chemical, physico-chemical and physical.
2.4.2.7.1. Biological methods.
They look at the chemical state of Na: sodium in the red cell, which changes
slowly with the medium of suspension (70); in the muscle there is a fraction of
sequestered Na, as in the oocyts of amphibians. In
humans, using the principle of Gerbrandy to determine
the fraction of bound electrolytes, it was concluded that 6-10% of plasma Na is
bound (233). This very small fraction is explained by the fact that the venous
stasis that accompanies the accumulation of H-ions, displaces Na from its
interactions with proteins. Other results show that the chemical activity of Na
and K falls, in the presence of protein as well as in the presence of
fibrinogen.
2.4.2.7.2. Chemical methods.
Their use requires a pre-separation of the cation
from other compounds in solution with which Na interacts. Chemical methods are
suitable for determining total Na in blood after precipitation and
centrifugation. Estimation is performed by titrimetric
methods. (86, 244).
2.4.2.7.3. Physical methods.
(a) absorption in ultraviolet, visible in the X-ray
(b) emission spectroscopy
(c) electroanalyitcal methods (122) including potentiometry determinations with ion-selective electrode
and voltammetry.
(d) isotopic
(e) nuclear activation
(f) chromatographic
(a) absorption spectrophotometry
and fluorimetry are based on the interaction of metal
and of compounds that contain it with electromagnetic radiation, according to
Beer-Lambert law expressing linear dependence of the absorption of radiation on
concentration of the substance. In principle fluorimetric
methods consist in the linear dependence between the excited molecules and
light emission in the course of returning to the fundamental state, according
to Stokes law. However, this requires chelating with organic compounds
preferably with aromatic compounds, forming fluorescent compounds. Chelating
and is also used in absorption spectroscopy. The methods require a prior
separation, the most widely used one being the methods of separation by
chromatography.
(b) emission
spectroscopy: Flamephotometry is a method of atomic
emission, the atom is excited by flame and the intensity of emission is
proportional to the atomic concentration. The Na emission lines are the D lines
with a wavelength of 5890 and 5896 A. The method is applied for determination
of total cations (Na and K). The organic substances
are completely destroyed by combustion. It has great value for clinical use.
Flame-atomic spectrometry. The biological specimen is atomized and excitation
is performed with a cathode lamp adjustable for specific wavelengths.
X-ray spectrometry uses atomic activation by means of X-rays and X radiation
emitted is recorded.
(c)Electro-analytical methods
(29, 74, 125, 142, 149, 150): Potentiometry with
ion-selective electrodes measures the plating cell potential versus the
reference potential "0" As a principle, the potential of the active
electrode versus the reference potential is proportional to the concentration
of active chemical species, selected by the ion-selective electrode similar to
the H electrode in pH determinations. The method is used for determination of
electrolytes in whole blood, undiluted plasma and other biological fluids (84).
The ion - selective method produces the value of the active form of ionized Na
and K mM /l, lower than values obtained by flame
photometry, either because of Na binding protein or the formation of ion pairs
(126). The precision of the method increases as the degree of dilution increases
and is maximum for the basic condition: infinite
dilution. The ion-selective electrodes can be used in vivo and in cell cultures
(35) enabling the study of the Na / K during cell function. Anodic voltammetry also uses selective electrodes versus the
reference, mercury electrode.
(d) The methods include isotopic
methods of analysis by dilution with isotopes.
(e) Methods of nuclear activation
(Neutron activation analysis = NAA). In this way the sample is irradiated with
thermal neutrons and emits radiation,
,
,
, which can be determined.
(f). Chromatographic methods also require preseparation of cationic with various means:
electrophoresis, precipitation, ultrafiltration, dialysis. Chromatography was originally used for the
separation of organic species and subsequently to metallic ions. Adapting these
methods for inorganic analysis was done by amending the two phases, stationary
and mobile, simultaneously or successively, as needed (125), for the monovalent and divalent cations. Chromatographic columns are made of glass or
metal (aluminum) filled with inorganic or unpolar
beds with different additions: tyramine, compounds in
the crown for excellent separation of monovalent cations, followed by photometry (150, 179, 200, 201).
Stationary phases may be liquid, cellulose, silicagel,
polyacrilamide, alumina, alone or in mixtures
adsorbent, inorganic ion exchangers (aluminosilicates
rich in Na, K, Ca, Sr, Ba
exchangeable with cations in the electrolyte
solution, hydrated oxydes, etc.) Organic on
exchangers: ion exchanging resins with varying degrees of acidity, resins of
cationic polymerization, basic resins, chelated, amphotere resin that have incorporated complex agents, or chelating
agents with a high capacity of selectivity, electrons exchanger resins, resins
with varying degrees of porosity, with polar groups which gives them hygroscopicity, by interacting with ions which alter their
Natural hydrophylia Na
K
Cs
Rb
Li. The stationary phase can be modified by impregnation
with polysaccharide ions: heparin (179 ), dextransulphates,
condroitinsulphates with sulphydrilic groups that set cations.
Heparin (considerably reduces the retention of anions), biliary
acids, etc. The impregnation of the stationary phase with special substances,
allows the separation of anions and cations also
according to their valence. The chromatographic separation enables one to
obtain values comparable to other methods. The eluent
can be chosen and prepared so as to allow selective elution also function of
the fixed phase, HCl, tartaric acid, etc. Among the
methods outlined, the vast majority do not meet the basic condition i.e., to
keep intact the structure of the composition of the environment including
macromolecules. The methods that use ion-selective electrodes meet these
conditions to the largest extent, being also used in vivo. They require special
attention for more convenient measurements. I presented the factors affecting
the values of the chemically active Na+(231, 232, 233).
Plasma Na+ is in interaction with a wide variety of anions and
anionic sites on macromolecular compounds and in conformity with the law of multiple equilibrium, the energy intensity variables.
2.5.
Interactions between plasma components.
2.5.1.
General.
The physical, chemical processes and the transport of substances in the blood
take place in conditions of constant temperature and pressure. These processes
are controlled by concentrations, structural properties and their reactivity
(162, 239). Substances dissolved in plasma are defined as ligands
(L), transporter (T) and disturbing agents (159, 188). Ligands
are soluble substances; they are very polymorphous, from electrolytes to
proteins (161). The transporters (T) are most often protein-bearing reactive
ionizing groups (12). They interact and are thus the conduct of their
physiological role is assured. LT interactions depend on: (1) the Nature of the
ligand, (2) the Nature and concentration of the
carrier, (3) their affinity and type of interaction, (4) mechanisms for the
release of ligand to the target, (5) modifying
components involved in the physiological and pathological processes.
From the point of view of 2nd law of thermodynamics,
transport occurs if there is a gradient different from "0" of one
parameter.
The thermodynamic force generates a stream (94), whose
final purpose is the destruction of the gradient. In living systems, however,
regeneration of the gradient occurs at the same time with the cancellation of
the gradient by means of general biological physical and chemical processes,
and by means of metabolic processes. The Natural processes involved in gradient
destruction are: diffusion, convection, osmosis, adsorption and absorption,
capillarity, etc. The action of mechanical processes and the flow of fluids
(blood, lymph) are added to those processes, speeding up the destruction of the
gradient.
2.5.2. Ligand/transporter
interaction (L / T) (101)
All the molecules attract one another from distances not compensated by
repulsion forces (92,218). The LT bond is uncovalent,
electrostatic, of low intensity, variable, nonspecific and therefore allows different
combinations. Strength depends on the anion - cation
distance, on for the number of opposite sign groups in the neighborhood, the
number of water molecules around the ions, their polarizability
(57, 56). The ligand observes the law of mass action
for a bimolecular system.
[L] + [S] => [LS] where [L] = ligand
[S] = site on the transporter: k = association, dissociation constant. The
equation to balance measures the affinity of L for S, which is defined by the
relationship:
K = [L] [S] / [LS]] = K / K
Determination of the constants K1 and K2 above in vitro,
requires very pure and stable ligands and in order
not to alter the data by the presence of nonspecific binding (231, 236, 167,
168, 224). One can use substances that allow the characterization of the
competing set of particular places. The ligand-transporter
association constant increases with temperature. There is an inverse
correlation between serum protein concentration and affinity. The
characterization of the physical-chemical interaction LT enables the
determination the number of reactive sites on the transporter and of the
equilibrium constant Kd.
It defines the number of coordination - the number of molecules attached to the
central ion according to the binding theory (Klotz, 112) which uses molecular orbitals and the theory of binding valence (10). Measuring
of the transporter ligand is done through various
methods: filtration, centrifugation, dialysis equilibrium (232). This method
has enabled identification of the Na chemical activity in the blood. The
criteria for specificity of LT binding are saturability,
reversibility, physiological and pharmacological specificity. Binding stereospecificity is a measure of selectivity.
Studies of saturation of the process of interaction,
enable the determination of binding parameters (218, 232, 233, 231, 224, 236): the density of binding sites (Bmax)
(92). The kinetics of the LT interaction is being investigated on the basis of
the law of mass action and of the Michaelis-Menten
equation. The binding constant and the number of binding sites (Bmax) are calculated with the Scatchard
equation (180):
B / F = Bmax-B / K (180)
The regression curve is right for a single set of
interaction sites on the transporter. Non-linearity indicates the existence of
several other sets of sites.
The role of LT interaction. The free fraction of the ligand
is biologically active. The LT association also operates as a place of storage.
In these conditions, the ligand is released gradually
according to the multiple equilibrium theory (Klotz) (112, 143) as it is
consumed in the target organs. Determining the two forms accompanying some
disorders in cases of abnormal transport has etiopathogenic
value. More and more data free is accumulated in favor of the need to
investigate the mechanisms of blood transmission of some substances: hormones,
electrolytes, suggesting the concept of blood transport pathology, the dynamics
of this process including the association and dissociation of the LT complex.
2.5.3. Types
of L / T interaction.
2.5.3.1.
Ionic bonds.
Ionic bonds are interactions (147) between alkaline metals and ionic groups,
especially the oxygenated ones, between which there is a partial transfer of
electrons from metal to nonmetal (101, 153, 159, 213).
2.5.3.2.
Hydrogen bond.
The hydrogen bond (218, 147) appears as an auxiliary valence of hydrogen
covalently bonded with a strongly electronegative atom (O2, N2).
The most frequently encountered are those with proteins that act as a donor and
acceptor of proton (12, 52):
Table
10: Types of hydrogen bonds (52).
-
O-H. ....... O - H
- O-H ........ O - C
- N-H ........ O - C
- N-N ,,,......N -
The Nature of the interaction is electrostatic, with an intermediate electronic
distribution between the mezomeric forms. Bridges are
usually the privileged directions imposed by the strongest interaction
corresponding to co linearity.
2.5.3.3. Van der Waals forces.
These are intermolecular forces of attraction between the fluctuating
electrical charges (153). Interactions are established: between groups with
opposing electrical charges as associations between pairs of ions forming
saline bonds or interactions between positive groups and chargeless
groups, or between polar groups without significant charge. Within the
hydrophobic inside of the protein molecule, there is a dielectric medium which
favors the polar interactions.
2.5.3.4. Electrostatic interactions.
Electrostatic interactions act alone or in connection with the bonds discussed
above. They operate between all molecules at a distance non-compensated by
forces of repulsion.
2.5.3.5.The London
forces of dispersion.
London
dispersion forces operate between molecules and are due to the electric field
of the dipoles with very short life induced by the nucleus-electron motion of a
molecule.
2.5.3.6. Physical forces of adsorbtion-desorbtion.
There are physical forces of adsorption / desorbtion
on the surface of serum protein. The processes have no curve of saturation.
2.5.3.7. The role of water.
Water plays an important role for development of transporter-ligand interactions (27). Water has a high dielectric
constant because of its hydrogen bonds between molecules. Its molecules combine
with ions and produce hydrates at the water dipole.
2.5.3.8. Cation -
association.
Association cation-
is a form of interaction of cations
with aromatic amino acids. It has been incompletely studied up to now, both as
a functional mechanism and in point of its functional significance as another
mode of transport for Na. Cation-
interaction - is formed between Na and the
orbitals
of aromatic amino acids. This interaction is uncovalent
(49). It was identified through crystallography in 1960. Cation-
associations are structural and functional aspects of static
and dynamic energy of the protein.
The compound is formed of the ligand
and the aromatic residues of the amino acid side-chains of protein. The ligands are cationic amino groups (68), alkaline metals,
etc. The structures involved in the interaction with cations
(Table 11) are aromatic amino acids or their residues from protein
compositions, phenylalanine, tyrosine, tryptophan, pirolic ring of globular macromolecules (90), acting as a
donor of electrons, and provides flexibility in environments with low polarity
and favor cationic access to imidazolic level,
preferred to form the cation -
complex with smaller distances than Van der
Waals forces. Cation -
interactions are also established with polar and hydrophobic
residues (48) between coordinated ligands to a metal cation. These coordinated ligands
interact with asparagine, aspartic acid, glutathione,
histidine, treonine, valine, (251), with two molecules of water (250), lisine (184). Aromatic rings have a well defined electronic
distribution whose
result is that cations are arranged perpendicularly
on a plane. The study of cation-
associations is performed with computational methods, with
mass spectroscopy, analysis of solid structures, crystallography, fluorimetry (emission of fluorescence analysis) of aromatic
nucleus in interaction with metallic cations (Na, Li)
(226). The mechanisms of
-cation interactions are multiple. Forces of electrostatic attraction correctly describe the
-cation interactions (48) between cation positive charge with quadrupolic momentum of the aromatic ring. Chemically,
there are also cation-
non-conventional interactions, where the electrostatic
attraction is achieved without an input of electrons (184). In some cation-
interactions there are phenomena of polarization deducted
from the effects of perturbation of the potential of general molecular
interaction. The polarization is accompanied also by electrostatic forces and
by dispersion-repulsion forces. Molecular interaction through polarization
enables the very quick binding of cations to aromatic
compounds (42). From the analysis of the fluorescence emission of the solution
containing aromatic amino acids and cations (Na, Li)
one demonstrated the deactivation levels of radiative
energy transition of the
orbitals
(226) and established that the bases order of anions is
indol
phenol
benzene
in the interaction Li-
when anions are reduced in the presence of a cation (96).
The role of
-cation association. This
association is a favorable interaction in biology (184) in various proteins
that interact with cationic ligands (250). They are
involved in molecular recognition (9, 40) - at the interface protein - protein
and stabilization and defining of the Native structure , (68, 157) in
conformation and stability of metal-enzymes (68) and the functioning of the
enzymes that have a metal coordination center (88, 68) and in the ion channel
structure (37, 42). Sodium is non-polarizable and
that is why it does not have a preferential relation to an aromatic compound. Its
interaction in this association is electrostatic and perfectly describing the
correlation between the self-regulator field of energy and the molecular
electrostatic potential (42). These interactions are accompanied by coulombic forces, the transfer of charge and Van der Waals forces and orbital
energy of molecules in which Na is merely a non-polarizable
particle (42). It also established contacts with four carbonyl oxygen of leucine, proline, and valine tryptophan and two water
molecules. In these conditions, Na has a binding character between migratory
carbonyls of valine and tryptophane
(37, 250). The association Na / phenylalanine is
characteristic to IP-cation interaction in biological
molecules. The phenyl ring has but a small contribution to these associations.
Phenylalanine derivatives have a higher affinity for Na (65). Association with tryptophan confers substantial stability in the medium with
low polarity (94). In these interactions, Na binds for electrostatic reasons,
perpendicularly to the plane aromatic compound.
Table 11. Ionic composition of plasma (68)
protein groups, electrolytes, free amino acids, aromatic
amino acids.
(1) (2) (3)
(4) (5) (6)
cation -
COOH CH
Na
Cl
aspartic
involved tyrosine
OH CHOH K
SO
glutamic in phenylalanine
CHO C
H
Ca
F
histidine
quilibrium tryptophan
CO C
H
Li
- lysine
multiple histidine
NH
C
H
- - treonină
- aspartic
=NH C
H
- -
cystine
- asparagine
CONH
C
H
- -
arginine
- treonine
CORNH - -
- - - valine
SH - -
- - - lysine
(1) =
hydrophilic radicals. (2) = radicals
hydrophobia
2.6.
Chemical activity of plasma electrolytes.
Research of X-ray diffraction (68, 153) showed that
dry NaCl crystals are formed by ion Na and Cl just like in solution. Ions in water can move freely due
to the dielectric constant of water. In a solution containing NaCl and polianions, there is a
free equilibrium between free Na
and Na retained around the polyanion
through various interactions. The free fraction is quantitatively less than
that measured by potentiometry or conductometry,
therefore it does not show the results of dissociating the
electrolyte (153), (the vapor pressure, cryoscopic point) which would explain the number of
particles resulting from dissociating them described by an equation of the type
NaCl


Na + Cl 
because there is no such equation, and also a constant k.
The chemical potentials”
” and”
” of the solution of NaCl (Debye-Huckel cit.153) are associated with their
concentrations by the equations:

=

+ RTI

c

=

+ RTI

c
, where c
and c
are concentrations of Na
and Cl
.

and 
are the coefficients
of activity needed to apply the equations above. The values
of the coefficients ranged from "0" and "1" value
"1" is reached at infinite dilution.
Activity =
x c (concentration)
= activity / concentration where, the activity is actually a
concentration of ion in solution.
From this data there results an apparent decrease of
the concentration of ions with opposite sign, by the attraction between them,
with solvent molecules or anionic sites of proteins, when calculating the
concentration, a value defined as " cation
activity." Electrostatic forces decrease the free energy in parallel with
increasing the concentration of salts and increase the coefficient of activity.
The notion of "activity" is used correctly instead of
"concentration" for electrolyte solutions, defining the actual
capacity of interaction of free reactive forms . The activity of blood cations is an incompletely clarified concept due to the
complexity of the environment that changes the free forms of cations. The values reported depend on the method used to
determine them: the elimination or modification of proteins or by the
accumulation of catabolic acids (121) or in the presence of purified proteins
(137) or by methods that preserve the structure and function of macromolecules
(228, 231, 232, 233) using competition of polyanion
heparin for serotonin, - a method accepted and recommended by Al.Monnier (see facsimile). The results obtained with this
method show that in the blood serum collected from different species, the
activity is evident only after denaturizing by mechanically stirring the serum.
According to this data, in humans and rats (normal) one cannot detect the
cationic activity of sodium. This is in interaction with blood proteins (Table
11).
2.7.The transport of some substances in the blood.
2.7.1. General.
A chapter of such an expanse requires a thorough
treatment, which is not our intention. The paper only aims to illustrate the
problem with some substantial examples
in support of the idea of the physiopathologic
significance and physiologic mechanisms of transport in the blood of certain
substances, thus suggesting the opening of a new chapter of physiology, to
explain the fundamental physical-chemical mechanisms at the molecular level.
2.7.2. Calcium. (68, 78, 204)
It is very well represented in the body. It has an important role in the
processes of neuro-muscular excitability, the
transmission of nervous influx, an antagonist of K and the sympathetic system,
in haemostasis, in body static and dynamic, the bone
structures of resistance.
Its distribution in the body is unequal. Of the total quantity of 1000 - 1500
gm., 99% is found in the bone, bound to the organic matrix of collagen, 1% in
tissues and 0.1% extra cellular and plasma :
Ca = 6Ca – Pr / 3 / Pr + Ca.
Binding to plasma proteins depends on their
concentration, environment pH, H antagonizing the binding of Ca .
Ca homeostasis is maintained by
digestion intake, elimination by the kidney and bone metabolism. Digestive
intake is reduced by prolonged treatment with antibiotics, in steatorrhea, the deficit of bile (hepatitis, cholestasia) that disturb the absorption of liposoluble vitamins, including those in group D, followed
by perturbation in protein synthesis intestinal transport of calcium (Calcium
bind protein (CBP). In this case, a chronic disorder occurs in the metabolism
of calcium. such as spasmophilia,
also interesting prevertebral muscles of the cervical
vertebra, involved in the upright posture (234, 235). Hypercalcaemia
is encountered in hemoconcentration, decalcification,
bone rickets, osteomalacia, hipoparathyroidism,
nephrophaty, etc.. It is accompanied by muscles hypotonia, constipation.
2.7.3. Magnesium.
It is also a chemical element bivalent in the same group with calcium,
unequally distributed in heart, liver, brain, bones, etc. Only 1% is present in
the extra cellular environment. 35% of it is bound by albumine.In
plasma and red cells, there is 1.5 - 1.8 mEq of
magnesium of which 1.3 mEq bound to proteins. Its
intake is under endocrine and vitamin control. Magnesium is involved, like
calcium, in muscle excitability.
2.7.4. Copper.
Copper (26) is involved in the processes of oxidoreduction
and brain function. 90% of blood copper is transported bound firmly to ceruloplasmine, a-glycoprotein. 34% of that protein, is
associated with copper. Under the influence of kelating
agents, it loses copper and is whitened. The strength of interaction is
different. Copper interacts with the carboxylic residues of the glutamic and
aspartic acids. Perturbation induced in the copper transport is expressed as hepato-lenticular degenerescens
(Wilson's disease) and psychosis.
2.7.5.
Iron.
On discussing the physiology and physiopathology (142) of iron, one must take
into account the processes of transport and storage. Ferric iron is transported
on transferrin (siderophilin),
a 
-globulin which binds 1.25 mgr iron / gram protein. Under
normal conditions, 30 - 40% of transferrin is
saturated with iron. The mechanism of fixing iron to transferrin
is regulated by "up" or "down" regulation controlled by the
amount iron in the body. The amount of iron in the body is 4 gm, stored on ferritin whose various Names refer to the number of iron
ions bound: apoferritin which has no iron, monoferritin with an iron atom, diferritin
having two atoms of iron and hemosiderin, the form
saturated with iron , 37%. The iron in plasma and interstitial iron is carried
entirely to the target haematopoietic organs, attached
to transferrin.
2.7.6.
Hemoglobin.
Hemoglobin is transported to the reticuloendothelial
system on haptoglobin, a glycoprotein of the 
globulin fraction from plasma, a polycatenar
molecule containing sugars 22.7%, 5.5% sialic acid.
Low values of haptoglobin are present in hepatic
failure and anemia.
2.7.7.
Oxygen.
Oxygen is transported through well studied, understood
reaction, on hemoglobin and can be correctly described by the dissociation
curve of oxyhemoglobin (78, 99).
2.7.8.
Serotonin.
Serotonin (219, 220), a biogenic amine derived from tryptophan is involved in the function of the nervous
system as a synaptic mediator. It has importance in carcinoid
pathogenesis. It is completely transported, under normal circumstances,
attached to platelets. In pathological conditions, its transport is perturbed
and 5HT is also present in a free
state in plasma.
2.7.9. H-ion. Acidic-basic equilibrium.
Metabolic processes going on in tissues generate H+
which affects the blood pH shifting it to the acidic side. Depending on the
intensity of metabolic processes, there are differences of pH of the efferent
blood of different organs. Difference of pH also exists between different
segments of the vascular tree. The pH of arterial blood is 7.4 and that of the
venous blood is 7.35. A constant concentration of H is maintained through two
main mechanisms.
In the first stage, the physical-chemical processes of
the blood buffer systems come into play for the maintenance of the acidic-basic
balance (68, 78) (Henderson-Haselbach equation).
pH
= pK + log [A
] / [AH]
describing the couples of acid/base buffers. There are four buffer
systems in blood: H
CO
/ NaHCO
: NaH
PO
/Na
HPO
: acidic Hgb./ Hgb
: Acidic protein / Na protein.
In the mechanism of maintaining the blood pH, the most
important role belongs to red cells and plasma proteins. Proteins intervene by
their lateral and masked amino acid residues. Thus in the acidic environment,
proteins accept a greater quantity of protons . At the level of elimination
organs, protons are dissociated from the protein and removed. This is the
second phase of mechanisms that maintain the blood pH. The concentration of
hydrogen depends on age, activity, nictemer, digestion
phase, etc.
2.7.10. Circulation of hormones with transporters.
Chemical and functional heterogeneity of hormones is manifested in the
mechanisms of transport in the blood (202). Some are transported in a free state while others
are bound to transporters. Between these two forms there is equilibrium. The
unbound fraction is biologically active.
The transporters are proteins in the blood, most of them isolated and well
characterized. (Table. 12).
Table. 12. Transporters hormonal blood protein. (221)
Protein transporter
MW fluidic
fractions number.
HSA ( hormone serum
albumin)
69.000 0
CBG
(corticosteroid binding globulin=transcortin) 52.000 26
AAG (
-acid glicoprotein )
410.000
42
TEBG (testosterone-estradiol binding globulin)
94,000 32
TBG (tiroxyn binding globulin) 88,000 71
TBPA (tiroxyn binding prealbumin) 500,000 0
SHBG (sex hormone binding globulin)
IGF-BP (insulin like growth factor binding)
2. 7.10.1. Growth hormone (GH).
The growth hormone (GH = somatotrop). It has 191
amino acid residues in its structure
(242). Its molecular weight is 21,500. It has two sulphuric
bonds. In the peripheral blood one can detect a series of oligomeres
of GH (up to minimum one pentamer ). GH acts through somatomedins. It is secreted by acidophilic neurotrope cells in the hypophysis
concentrated more on the lateral sides.
Regulation of
GH secretion is achieved through the nutritional status and the stress of
organisms, of which the most important is the level of cellular proteins.
Malnutrition stimulates by feedback (60.14) the secretion of growth hormone
through hypothalamus with a regulatory loop that includes somatoliberine
and somatostatine. At the hormone receptor level
there occurs the separation of the polypeptide hormone
from its plasma protein transporter. The molecular weight of the receptor is
somewhat smaller than that of the original isolated receptor, the difference
being given by glycosilation. Anomalies of the
complementary DNA encoding for GH receptor are found in children with Laron dwarfism (syndrome of resistance to GH), proving the
biological importance of the receptor.
Protein binding GH.If radiolabelled
monomeric GH is incubated with human plasma, it
produces two forms of high molecular weight, due to the presence of a protein
binding, which is identical to the extra cellular domain of human growth
hormone receptor. The absence of the binding protein from the plasma of Laron dwarfism patients who are running a deficit of GH
receptor supports the existence of the relationship between protein binding of
GH and receptor. 45% of GH has the molecular weight of 22KD, of which 20%
complexes the binding protein and 85% is
involved with the regulating protein with great affinity. When the
concentration of circulating GH highly exceeds the value of 10 ,20
g %o a relatively smaller proportion is linked to the
transporter protein. Protein-bound GH is metabolized differently from the monomeric GH: it persists for more than 10 times in plasma
and its volume of intravascular distribution represents twice the compartment,
while monomeric GH is present in the extra cellular
space. These two factors may increase the biological activity of hormone
binding, but are counteracted by the competition for binding between receptor
and circulating protein. Plasma protein concentration is constant for each
individual. Plasma protein is higher in childhood and decreases with age.
It increases during slow wave sleep and during the
intake of free fatty acids. It also increases during fasting in anorexia
nervosa, cirrhosis. It decreases in obesity, the emotional disorders (emotional
depression). GH stimulates skeletal growth, the growth of connective tissue, of
the muscles, of the viscera. There are two hypotheses concerning GH actions:
the first one admits a direct action on cells, the second insists on somatomedinic mechanisms, attributing a special role to
IGFI,
2.7.10.2 Somatomedins.
Are represented by a group of polypeptides with low molecular weight
(6.000-8.500 D) with insulinoid actions. Somatomedins are produced under the influence of GH. They
are transported in plasma, bound to large protein molecules . Two proteins with
insulin structure were isolated from the blood: IGFII and IGF1 (insulin like
growth factor). The molecular weight of IGFI is 7000 D, its izoelectric
point is 8.2. Its proinsulin amino acid sequence is
formed from a small chain polypeptide (70 amino acids residue) This somatomedine differs from proinsulin in the C peptide: IGFI has a sequence of 12
amino acids while proinsuline has 35, with a different
sequence between sequences 8 amino acids and 12 amino acids. At this level, proinsulin is cleaved before secretion, while the IHFI
cleaves after secretion. IGFI synthesis is probably performed in the liver. 85%
of IGFI is carried by a protein with GM = 100,000 and the rest by another
protein with G.M. 50000 = D. Synthesis of the first transporter protein is
dependent on Chin the event of a deficit of GH, this protein is replaced with
protein MW = 50.000.The IGFI binding protein is identified as IGF - BP and can
be used as an indicator of the secretion of growth hormone in acromegaly. Circulating somatomedine-transporter
complexes are inactive and a separation process occurs in order to cross the
capillary wall in the interstice and from here to the target cell. They appear
early in the intrauterine life (week 20) when GH is not yet synthesized. IGFI
receptors are already present. This decreases sharply after birth, in maternal
blood. Somatomedins regulation is by GH, especially
for IGFI. Adjusting secretion of hypophysary GH is
achieved by inhibition of somatoliberin and
stimulation of the release of somatomedine. Estrogens
decrease IGFI concentration in normal subjects and in acromegaly.
The biological effects are related to IGFI binding to cellular receptors on condrocytes, lymphocytes, adipocytes,
fibroblasts. IGFI has tyrosinkinase properties, which
IGFII does not have. Somatomedins are fundamental
factors for the growth of cells, they mediate some cellular actions of GH.
They influence protidic and lipidic metabolism and, like insulin, stimulate the
transportation of glucose and amino acids into muscles, but by different
mechanisms than those of GH (36). They stimulate the formation of the organ and
bone matrix, capturing sulphate and thymidine in condrocytes whose
growth they stimulate. The somatomedinic hypothesis
starts from the observation that the growth of the mitotic cartilage in vivo
depends on the presence of GH inactive in vitro. It is known that IGFI and
IGFII are components of the serum. IGFII is increased in acromegaly
and low in growth hormone deficiency, suggesting that IGFI is the main mediator
under the influence of GH, the locally produced IGFO contributes to the
stimulatory effects of GH in particular to longitudinal growth.
2.7.10.3. Thyroid hormones.
They are iodate derivatives of tyrosine: tetra and triiodothyironine which have thyreoglobulin
as a precursor. Thyreoglobulin is present in
colloidal thyroid follicles and plasma. Thyreglobuline
is a glycoprotein. Electrophoretically it migrates
with albumin. Thyroid hormones are transported in plasma almost entirely in a free state: T
in a ratio of 0.04% and T
in a ratio of 0.4% are bound. The unbound
fraction is active on target cells and is operated by a feedback mechanism.
Transport in the blood is important both to hormones (203)and plasma iodine
which is organicized in the thyroid gland. Iodine is
carried by the transport protein of iodine (iodine binding protein = PBI). Iodine
is carried actively in the thyroid through a mechanism of sequestered iodine
pump. Its content gives indications about circulating hormones. There are three
T
binding proteins in the plasma: thyroid hormone binding
iodine (TBI) (tb.nr.12). It is a glycoprotein monomer, with a concentration of
1-2 mg /dl, and a half-life of 5 days. It has only one binding site for T
and T
. It carries 70% of
thyroid hormones for which it has high affinity. In electrophoresis it migrates
between 
and 
-globulin. TBPA with plasma concentration of 25mg/dl with the
half-life of 2 days. It carries only T
.
It carries only T
. It has less affinity for the hormone. Carrying 20% of T
. TBPA is synthesized in the liver. Albumin and prealbumiNa carrying 10% and 30% T
, T
. Capacity binding protein is influenced by certain
conditions, and especially the TBG. Increased protein decreases the level of T
and temporarily
increases secretion of hypophysial TSH , therefore
the synthesis of T
and T
At the same time with modifications of binding protein
concentrations or their capacity to interact with hormones there occur changes
of hormones concentration. Converting T
to T
is dependent on deiodinase in the
pituitary and liver. There are a multitude of(242) conditions in which TBG may
modify its ability to bind thyroid hormones. T
is deiodinated at T
in peripheral tissues: liver, kidney, brain, thyroid. HT level (198) is not influenced by age though the
proteins are modified: albumins concentration decreases and there occurs a high
concentration of
-globulins. TBG synthesis is accompanied by increased blood
lipids that inhibit the binding of proteins to T
transporter, TBG, TBPA and prealbumins,
a process, called "thyroid hormone binding inhibitor = THBI. Thus, T
binding decreases in the elderly who have a poor state of
nutrition. THBI increases its activity if the TBG and albumins decrease. It
therefore lowers the concentration of bound T
and increases the free form especially if chronic
respiratory, kidney, cardio-vascular diseases are present and accompanied by a
decrease in T3 as well.
Adjustment of thyroid function is performed by
classical mechanisms involving the thyroid, hypothalamus, pituitary and
peripheral consuming hormones, with a feedback mechanism. HT complexity of action,
with the associated transport mechanisms, requires in addition to clinical
activity of the thyroid gland the highlighting of quantitative aspects by
determining their concentration and the
investigation of transport mechanisms through several methods:
(1) Determination of iodine-related PBI.
(2) The analysis of state of competitive binding by determining competitive
dislocation of T4 from TBG.
(3) Radio immune methods.
(4) Measurement of serum T4 and T3.
Dosing of T3 has no meaning, its values are always normal in the
plasma.T4 values (70-150 mM% a) reflect
binding forms.
(5). Dosing the quantity of free hormones by equilibrium dialysis method,
against a buffer, in such a way that only the free hormone can dialyze. From
the dial sate one determines the hormone radioimmunologically.
The method has heuristic value.
(6) Analogue methods analogous. An HT antibody is
added and one determines a derivative marked by HT (analog), which binds de
-antibody and not the binding protein of T. Competition
between the analogue and the free HT allows determination of free HT.
(7) Immunoanalysis in two stages: first, free HT is
extracted by fixing on an antibody and in the second phase free HT marked on
the antibody unoccupied sites is measured.
.
2.7.10.4. Corticosteroid hormones.
Corticosteroid hormones are somewhat similar
mechanisms of transport (246). The adrenal cortical has three distinct
morphological, enzyme and secreting areas: the glomerular
area secretes mineral corticoids (aldosterone, desoxycorticosteron): the fasciculated
area secreting glucocorticoids (cortisol
and corticosteron): the reticulata,
which secretes sexoid hormones (dehidroepiandosteron,
androsteron, oestrogens).
Their blood levels vary with age, nictemer, the level
of physical activity, etc.. Adrenal cortical hormones are derivatives of
cholesterol (215, 216) with progesterone as their turntable. Adrenal cortical
hormones, as in fact all steroids, are transported in the plasma, most of them
protein-bound (54). A small fraction of bind to circulating red cells and a
lesser part are in a free state.
20% of aldosterone and 5% of the amount of cortisol are associated with red cell. The proportion
related to red cell is influenced by the concentration of total blood. Protein
transport of corticosteroids (tb.nr13).
Table No 13. Transport of major corticoids (78)
Hormone %free %albumin bound %bound CBG
Aldosterone 37 41 22
Cortisol 4 6 90
The adjustment of cortisol
secretion recognizes the general feed back mechanism having as the starting
point the concentration of free hormone. In humans, CBG binds up to 25
g / gm. As CBG becomes saturated, binding passes on to
albumin. The adjustment of mineralocorticoids
secretion has a more complex mechanism, involving, in addition to the common
stimulus - the concentration of free hormone in blood- the level of extra cellular fluid and of the
blood, arterial pressure, Na and K concentration in plasma correlated with the renin-angiotens in the system. The corticosteroids
pathology recognizes as a mechanism,
their synthesis, concentration and quality of plasma protein transporter,
mainly CBG, (80) and finally the relative strength of the tissue toward the
hormone, thus lowering their use. Structural changes in quality and reactive
protein transporter works by increasing the processes of dissociating the
complex CBG-cortisol. CBG activity is low in some
types of stress (bacteria shock, fungi, or surgery, burns, operations in the
abdomen and thorax, etc.).
Hypoalbuminaemia is also accompanied by a decrease in bound cortisol. These data confirm the importance of binding and
transport mechanisms in the pathogenesis of endocrine disorders interesting the
glucocorticoids. These processes are difficult to
highlight in the case mineral corticoids processes, as binding and dissociation
are fast and the hormone is bound for
less time. Adrenal sexed hormones have mechanisms of transport and
binding similar to those of gonadal hormones. They
are bound by SSBG glycoprotein (tb.12) and albumin for which they have lower
affinity.
2.7.10.5. Gonadal hormones.
Their synthesis takes place through the same mechanisms as those of other
steroids. Synthesis has acetyl coenzyme A as a starting point (216). The
sequence is: mevalonic acid, cholesterol,
progesterone and finally steroid hormones.
Male gonadal hormones: testosterone is secreted by
the Leydig interstitial cells together with androstendion and dehidroepiandrosteron.
Plasma concentration of testosterone in adults is 0.65
g/ml, of which 23% is bound. Androstendiol
has a concentration of 100
g/ml. And dehydrotestosteron
concentration is of 30
g/ml (23).
Testosterone circulates in a bound state, bound 100%
to a β globulin, TEBG, having low capacity and to SHGB, together with dihydrotestosterone and androstendiol.
Synthesis is driven by oestrogenization. TEBG is
synthesized in the liver. It is generally admitted that senescence of the
endocrine system refers to the ability and rhythm of glandular secretion, to
the transport and metabolism of hormones in regulating the activity of systems
and hormonal receptor sensitivity (88). Another part of testosterone (37%)
circulates in a bound state, related to albumin, and a significant part is
carried on CBG. Only 2% moves in a free
state.
Beyond the age of 60, the plasma free fraction
decreases and the albumin-bound fraction increases , due to the growth of
increase its affinity for steroid. There is also an increase of affinity for
SHBG, whose synthesis is stimulated by oestrogenization
in the elderly.Progesteron however, induces a
decrease of testosterone bound fraction. The metabolism occurs in the liver.
The specific action of testosterone is to stimulate spermatogenesis.The
non-specific effects refer to secondary sexual characteristics and metabolic
effects. Secretion adjustment is achieved by feed back with a hypothalamus and hypophysis link acting through LH and FSH gonadoliberines and goNadotrophins.
Female gonadal hormones are secreted, as other steroids, by glands
of coelomic origin, the ovary which has three
anatomic functional compartments: follicular, secreting mainly, oestrogen hormones (oestradiol
and oestron); progestational,
composed of lutein cells and thecal
cells which have progesterone as a specific product, and stromal,
made up of stromal cells secreting androgens (androstendion, dehidroepiandrosteron,
etc.).. The ways of synthesing them
are the same as those of all steroids (215, 216).
Oestradiol is transported bound to SHGB, albumin, and the CBG.
Together with oestrone, it binds, in a proportion of
98 - 99% to particular sites, with strong interactions, and thus it regulates
its activity also owing to the multiple possibilities of the ovary to synthesize
oestrogen hormones. Due to this fact, their
concentration is not relevant, and, in addition , it intervenes in the response
capacity of tissues. Ovarian hormones circulate mostly bound to albumin and
globulin transporters. SHBG has a small binding ability, but has high affinity.
Oestradiol, progesterone and oestron
bind to a lesser extent. In the elderly, cirrhosis and hypothyroidism patients,
SHBG increases in parallel with the oestrogenisation
of the person. SHBG concentration in plasma is 2-3 mg ‰. It is synthesized in
the liver. Synthesis is stimulated by oestrogen and
inhibited by androgen. Progestern and
17-hydroxyprogesteron are transported also on CBG. Albumin has little affinity
for these hormones, but their binding capacity is greater than that of THBG.
Ovarian hormones are distributed among various adipose tissue, digestive
tissue, target organs: endometrium,
myometer, brain, mammary tissue, kidney). The free
forms of ovarian hormones have a catamenial rhythm.
They are eliminated by the kidneys and in bile. Their action is specific on the
target organ and unspecific on metabolism general. The transport of
progesterone uses the same transporter as oestrogens
(173). Its synthesis increases during pregnancy. Progesterone has an oestrus cycle. Metabolism takes place in the target organs
as well. Its main activity is to differentiate cells of progestogen
organs.
[The remainder of Chapter 2 will be
featured in the upcoming November-December issue of this Journal.]
Professor Marcel Uluitu, M.D. Ph.D. began his
scientific activity in Physiology in 1953 at the Physiology
School (Medical
School) Cluj-Napoca,
in Romania.
He continued his scientific activity in Physiology in Bucharest at the
Institute of Physiology and Pathophysiology Daniel Danielopolu until 2004, at
which time he retired as Director of the Institute; he had held this position
since 1990. His research work includes studies of the central nervous system
physiology relating to the transmission of information in the nervous centers;
cerebral excitability, using methods, in their evolution, from the
determination on reactive isolated organ, to the most complex physical and
chemical methods, in the present. In his research of chemical mediation he
studied acetylcholine, serotonin, and their connection with the cerebral
metabolism.
Professor Uluitu has also investigated
cerebral tissue excitability, studying the structure modification of the
protein macromolecules, and the physiological and pathopysiological processes
in which are involved Sodium and Lithium. He implemented an original method for
physical and chemical processes which involve the chemic active sodium, in
normal processes and in the cerebral excitability dysfunctions, in human and in
experimental model (animal). These results of this work gave him the chance to
outline the chapter herein relating to the physiology of substances transport
in the blood. This is based on the physical and chemical interaction between
blood components.
His papers are included in the
collections of the U.S. National Library of Medicine and the U.S. National
Institute of Health. He is a member of the Romanian Academy
of Medical Sciences.
Dr. Diana Popa (Uluitu) is a
researcher in the Department of Microbiology, Immunology and Molecular Genetics
at the University of Kentucky in Lexington,
Kentucky, USA.
She attended Medical School at Vasile
Goldis University
in Arad, Romania,
and graduated in 1998; during summer breaks at medical school she volunteered
at the Institute
of Physiology D. Danielopopu
in the physiology and microbiology laboratories as well as in the clinic. After
completing medical school and a one year internship, she worked as a Research
Assistant at the Danielopopu Institute for three years before coming to the University of Kentucky. In addition to her medical
thesis, which focused on the actions of Lithium on the central nervous system,
since coming to the United
States she has published numerous additional
papers relating to Lithium and other substances. In continuing her on-going
studies, Dr. Popa is currently pursuing a Master’s degree in Public Health at
the University of
Kentucky.