The
Physiology of Transport Substances in the Blood (Sodium)
By Professor Marcel Uluitu, M.D. Ph.D.
Spiru Haret University
Bucharest, Romania
Co-Authored by Diana Popa (Uluitu), M.D.
Department of Microbiolgy, Immunology
and Molecular Genetics
University of Kentucky, Lexington,
Kentucky, USA
[Editor’s Note: This paper is presented as Part I of a
series of chapters from the new book “The Physiology of Transport Substances in
the Blood (Sodium)”; subsequent chapters will be featured in upcoming issues of
this Journal. This segment features Chapter One (of six chapters) and Section 1
of Chapter Two]
MOTTO:
Measure what is measurable
and make measurable what is not,
-- Galileo Galilei (from Ev.Z. 2008)
Preface:
General mechanisms
regulating functions: Body unity,
control and coordination of its functions are achieved by means of nervous,
humoral and physico-chemical mechanisms.
The nervous path is fast and turns on as a result of the analysis and control
of the functioning level of organs and tissues.
The humoral mechanism regulating the functions of the
body recognizes the transport of substances as a means by which it provides for
the needs of every tissue metabolic activity, synthesis of own substances,catabolites removal, etc. The humoral pathway is enabled as
a result of the analysis by the nervous system of the internal environment,
through feedback mechanisms, of the level of normal concentrations of
constituents of the body and their balance within the three spaces, including
nutriments, new compounds synthesized in some specific tissues, catabolites,
etc. Humoral pathway activation mechanisms depend on the role of these
substances in the economy of the body (composition, physico-chemical structure,
functional role, the energy structure of the body, etc.).
The physico-chemical pathway includes the body
composition and the circulating and structural substances which interact by
means of trace energy and have significance in the processes of transport in
the blood. The physico-chemical route of regulation depends on the composition
and structural-functional lability of proteins. These substances are in
constant motion, ensuring a local and global uniformity.
Transport of substances in plasma is in a state of
trace energy interaction ionic, electrostatic interactions, Van der Waals
forces, hydrogen bonds, London
forces of dispersion, cation-pi interaction, etc. These chemical interactions
decrease the activity of electrolytes and of other compounds. The result of
these interactions is a ligand-carrier complex whose
unbinding is
subject to mass action law.
Disorders in the substance transport mechanisms in the
blood generate a special pathology whose recognized causes are deficiencies
(anemia ,acidosis, etc.) ,genetic disorders (Wilson’s disease involving
Cu),endocrine diseases involving Ca ,abnormal excitability neuromuscular
disorder, behavioral-type constitutional disorders (involving the transport of
sodium).
Chapter 1
The
General Structure of the Body.
Transport
of Substances in the Blood.
Sodium is a chemical element in Group I
of the periodic table. Very widespread in Nature, it is at the same time the
monovalent cation best represented in the blood. It is extensively studied in
both kingdoms because of its association with multiple processes.
It is associated with metabolic and enzymatic
processes acting as cell activator. It is mainly involved in the cell membrane
functions. It is an extra cellular cation and is present more especially in
blood. In the excitable cells, the action potential is also identified under
the Name of sodium potential. It is an activator of the heart, in vitro. It has
an important role in the electrical manifestations of the excitable membranes
of cells; neurons, cell rods, excitoconductor cardiac tissue, etc.
At the synapse level it acts in
conjunction with acetylcholine. A seriously low concentration of sodium is not
compatible with life. Sodium blood homeostasis is assured by a very complex
regulatory system starting with food intake and continuing with transport in
the blood, sodium distribution, and elimination by the kidney, the digestive
tract, the skin, etc. Such mechanisms are nervously and hormonally regulated
(adrenal cortical, natriuretic hormone), osmoregulatory mechanisms, mechanisms of cellular and molecular emergency. In case of
acute intake increased, sodium is retained temporarily in the connective
tissues, interstitial tissue and fatty tissue in order to maintain sodium
concentration.
In the cell membranes, it works through
sodium ion channels interacting with the anionic sites of the proteins in their
structure. Thus, the information conveyed by the cation depends on the local
ion concentration and on the size of their ionic radii, varying according to the
number of hydrating water molecules. Its action can be antagonised in the ion
pore region of the Na channel, by neurotoxines.
Its passage through cell membranes depends on co-transporters that have,
however, a small importance compared to the passage using the ion channel and
gradient mechanisms. Sodium has an insignificant role in the genesis and
maintenance of the resting potential. These processes depend on the restoration
metabolism and on potassium. Na+ is not involved in the functioning
of non-excitable membranes. It is a vitally important cation. One more reason
for this is its value as a biological constant of the blood.
The regulation of sodium activity in
the blood depends on its interaction with proteins. Sodium chemical activity
determines the capacity of response of nervous structures by the generation of
an action potential. The fraction of chemically active sodium in the blood
highlights the role of physical and chemical interactions in the body.
Determination of total blood sodium with classical methods (physical, chemical,
etc.) after removal of macromolecular organic compounds has the same value as
the basic analysis of the composition of living organisms. It is a sort of
"Chemical anatomy." The physiological role of Na+ can be
established only by determining its chemically active form while maintaining
the full composition of plasma. There are multiple interactions among plasma
constituents. Despite the small energy values of such interactions, their
number is particularly important.
The method for determining the chemical
activity of blood Na+ described in this monograph has as the
reference system the energy of interaction, E
, between serotonin (5-hydroxitriptamin = 5HT) and the
polyanion heparin (Natural components of blood). If there is Na+ in
the solution, whose interaction with heparin is accomplished by energy E
E
, the interaction 5HT-heparin no longer takes place and 5HT
goes out of the dialysis pouch
The protein anionic groups present in the blood do not interact with serotonin,
(as this is Naturally transported by platelets), but they diminish Na+
interaction with heparin in a similar manner with the one in the cation
hydration process described in the text and induce E
values that are lower than E
, thus conserving the interaction of heparin with 5HT. The intensity of such interactions depends on the structure and
function of protein in solution. Because Na
affinity for heparin is limited by interactions with other
plasma compounds (proteins), the amount of dialysable 5HT decreases
proportionally to the number of molecules bound to heparin. Serotonin is not
appropriate for a blood test because it is attached to platelets that are the
amin Natural carriers. It was thus established that plasma Na+ has a
very low chemical activity both in humans and in laboratory animals (rats).
Individuals presenting such a picture have normal excitability.
In the normal groups of both species
studied, there are subjects in which the chemical activity of cations (Na+
up to 95%) antagonizes the interaction between heparin and 5HT, the latter
leaving the dialysis pouch.
Prof. Alexandre Monnier (biophysicist
at the Sorbonne) said that throughout his entire life he strongly believed that
Na+ is circulating in the blood in a state of interaction with
proteins, but he never had a method to prove it. The statement is repeated in a
letter presented in facsimile.
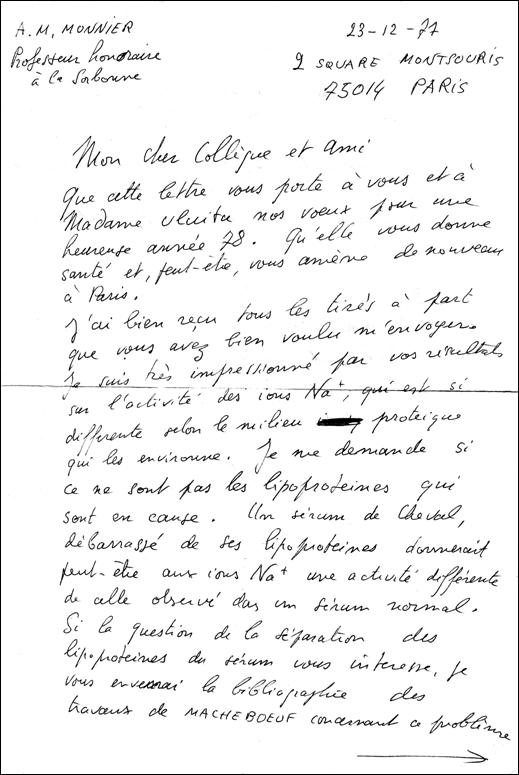
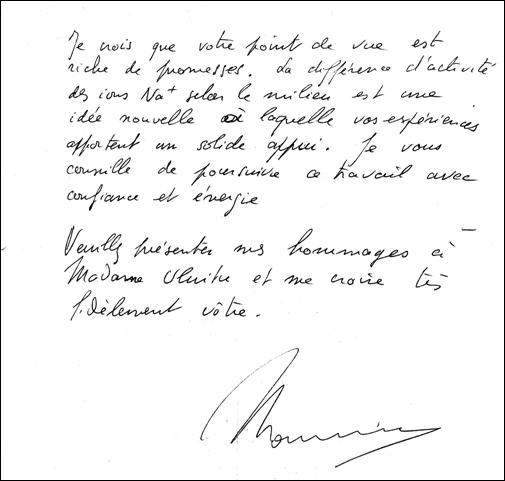
Individuals in whom Na+
antagonizes the reaction heparin/5HT present with increased neuro-muscle hyper
excitability expressed in the form of behavior disorders of a constitutional
type in humans, state of alert on EEG, poor concentrated attention, good
distributive attention, disorders in the regulation of the cardiac-homodynamic
function, while rats are prone to audiogenic convulsions, hyper mobility in
open areas, exaggerated intake of Nail, mineral-corticoid function deficiency.
The body can be divided into
interdependent compartments in point of transport mechanisms: INTRAVASCULARLY,
INTERSTITIAL ENDOCELULAR.
This division suggests the existence of two major
types of transport of substances: transport of the substance within the same
space (blood, interstitial, intracellular) and another transport between the
three COMPARTMENTS, across separator walls consisting of semi permeable
membranes and epithelia. The intravascular space communicates through capillary
walls with the interstitial space and the latter with the endocellular space
through cell membranes. Transmembrane transport, well-studied in both living
and artificial membranes takes place under multiple influences: blood pressure,
osmotic pressure and colloid osmotic pressure, concentration gradients,
electrochemical gradients, etc.
The term "space" includes
multiple interdependent structures, having close embryonic origin. The
embryonic origin of interdependence is preserved by the chemical composition
whose origin is the secretion of macromolecules in the cells of every
compartment. Macromolecules are secreted by vascular endothelial cells and by
other host tissues as well. Thus, a local, complex functional
structure is created. The structure acts in an integrated manner. This phenomenon
is known to organize and include the three spaces. In each of the three
anatomical structures the three spaces communicate among them. The chemical
composition of the compartments is maintained constant within the general
concepts of "internal medium" and "homeostasis". In this
context, the segment medium is maintained constant by the transport of
substances between the compartments. The substances in the three spaces have
oligoenergetic interaction. The complex connection between compartments is mediated
by semipermeable mentioned structures. The knowledge of the physical and
physico-chemical force enables a more precise understanding of the fundamental
state of structural elements and their functions. The following will deal with
transport processes, mainly in the blood, with particular focus on the
relationships between transport of Na+ in the blood and functional
status of the excitable and unexcitable structures.

Chapter 2:
The
Blood-Vascular Space
2.1. The Capillaries.
The
vascular tree is made up of the arteries, the capillaries and the veins. The
vascular wall (artery) is made up of three layers: the outer layer - tunica
adventitia, the second layer - tunica media, consisting of elastic and smooth
muscle fibers and, finally, tunica intima. Typically, the capillary wall is
made up of only a unicellular layer of endothelial cells which is surrounded by
a basement membrane on the outer side. At this level exchanges are taking place
between blood and the extra vascular space. It is an active physical and
chemical space separated by a cellular layer and an extra cellular layer having
a restrictive selective action for interstitial and blood components. For every
organ and tissue there are structural and functional features of the
capillaries that control the local exchanges.
2.2. Blood.
Blood is
the circulating tissue that irrigates all body cells. It circulates in a closed
system at variable speed given by the activity of heart pump, the elasticity of
vessels and size variations in different parts of the tree. Blood is composed
of a cell system (red cell, white blood cells, platelets) associated with
respiratory functions, antimicrobial defense, haemostasis and a liquid
intercellular system where the cells are floating – the plasma. Between the
dissolved substances in the blood there are chemical, physical interactions
resulting in the particular distribution of such substances. One should note
leukocytes and platelets contain 5-60 times more amino acids than plasma and
erythrocytes. Serotonin is also virtually wholly carried on platelets (219,220)
The blood tissue communicates with the
extra vascular interstitial space and through it with all body cells,
sustaining them and participating in the regulation of their function. In their
turn they affect the biochemical composition and physico-chemical properties of
the blood. This maintains the chemical and physiological homeostasis of the
inner medium within limits compatible with life. Blood plays a key role through
its circulating function as a transporter and participates alongside the
nervous system to maintaining, adjusting and adapting the body functions.
The total volume of blood is 5.5 liters
in adults, of which 3.5 liters (55%) is represented by plasma, and 45% of
volume contains blood cells (haematocrit). The volume of blood varies with sex,
age. The haematocrit varies with physical effort, environment temperature,
altitude, age of pregnancy, etc. The color of blood is red, with shades
depending on its gas content and Nature: oxygen, carbon dioxide, carbon oxide-
accidentally. Density varies with gender (1061 in men and 1057 in women)
consistent with haematocrit values. Viscosity, the resistance against the flow
by friction with the neighboring areas of blood components and the vascular
wall has values of 4.7 to 4.4 in men and in women, respectively.
2.3. Blood plasma
Plasma represents the liquid phase of
the blood. It is a transparent, slightly yellow solution wherein blood cells
are floating. It is a complex solution with heterogeneous composition of
inorganic and organic substances which are interacting among themselves, with
the solvent (water) and the vascular wall and their components. The solvent and
the compounds with low molecular weight are in balance with the substances of
the same kind in the extra vascular space.
2.3.1. Composition of plasma
Table 1. The classification of different substances in plasma
(17).
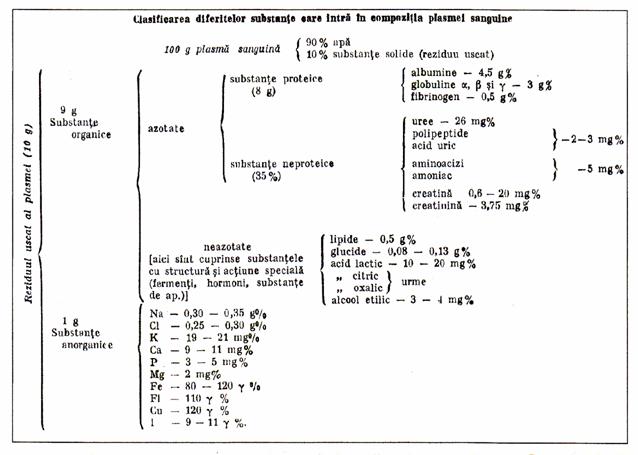
2.3.2 Plasma properties
Plasma is a real solution of crystalloids and soluble macromolecules. It has
some characters of a colloidal solution conferred by macromolecules, expressed
in that it does not pass through the membrane, the Tyndale phenomenon, without
expressing a condition of colloidal type in solution. The macromolecular substances
in plasma, in particular proteins, are amphoterious, having anionic and
cationic groups which interact with ions. The chemical composition of plasma
presents variations from one organ or tissue to the other according to their
functional or anatomical structure and their role.
2.3.2.1. Temperature
Organs with intense metabolic activity
(permanently or occasionally) have intense thermo genesis and efferent blood
temperature is higher. This increase in temperature affects the stability of
plasma solution and therefore the physical properties of plasma. Blood
temperature is also variable (37.7 C - 40 C) in various organs, depending on
the intensity of the metabolic processes specific to their function (liver,
brain) or on functional requirements (muscles).
2.3.2.2. Density and viscosity.
The lower density of plasma (1027) is
due to the protein. It is low in hypoproteinemia (hepatitis, nephropathy with
intense albuminuria, inanition, consumptive diseases).
Viscosity is defined by Newton as resistance to
slipping between adjacent layers, in the case of plasma between macromolecules
and between the latter and the vascular wall surface. It expresses the forces
of cohesion and adhesion that oppose blood flow and contribute to peripheral
resistance together with the vascular factor. Viscosity value is 1.86. The
viscosity coefficient”
"depends on the concentration of proteins. The viscosity
plasma is identified as "abnormal" or "structural" because
viscosity is not subject Newton’s
laws.
2.3.2.3. Osmotic pressure
It is a colligative property (68)
representing the force that the particles of the solvite are exerting on the
vessel wall. The chemical composition of blood varies between certain limits in
different regions in point of concentration of protein, gas (O, CO, etc.) H,
various electrolytes, some catabolites resulting from the tissues activity, etc
Osmotic pressure is best seen in a semi permeable membrane that separates an
aqueous environment (pure solvent) from the solvent solution. Its value is
given by the number of particles in the solution, not their Nature. Osmotic
pressure is described by Van t'Hoff equation:
v = niRT, the
ni = number of particles dissolved.
= Osmotic pressure.
R = gas constant.
T = absolute temperature
Another property of a colligative
solution depends on the concentration
of particles in the solution. This property is the "freezing point"
or "cryoscopy point."
Table. 2: Cryoscopic point value in different tissues
(129).
Tissue
|
Cryoscopic point
|
Blood
|
0,55 C - 0,57 C
|
Lymph
|
0,59 C - 0,62 C
|
red cell
|
0,50 C
|
Brain
|
0,65 C
|
Liver
|
0,97 C
|
Kidneys
|
0,94 C
|
Osmotic pressure varies with the
intensity of tissue metabolism. It is 6.7 atmospheres (matching cryoscopic
point is 0.560 C) and is given by the concentration of particulate
substances (dissociated plus non dissociated) in plasma: Na, K, Ca, glucose,
etc.. Under normal circumstances this is given, up to
90% by Na. Osmotic pressure is a condition for cell division in the processes
of excitability.
2.3.2.4. Colloid-osmotic pressure.
The plasma colloid osmotic pressure
(PCO) is produced, in addition to the substances mentioned by dissolved
proteins. They do not pass through the capillary membrane. Plasma protein
content is 2 -3 times higher than the interstitial content (7-8 gm vs. 2-3 gm).
The contribution of proteins to promote PCO is unequal as shown in Table 3.
Table 3 The value of PCO induced by plasma protein
fractions (78).
protein
|
molecular weight
|
gr%
|
PCO
|
albumin
|
69.000
|
4,5
|
21,8
|
globulin
|
140.000
|
2,5
|
6,0
|
fibrinogen
|
400.000
|
0,3
|
0,2
|
Total
|
|
7,3
|
28,0
|
At the level of capillaries, local forces are acting
that create a slight pressure imbalance according to Table. 4
Table 4 Average expulsion forces of the capillary liquid (78).
2.3.2.5.
DonNan’s equilibrium.
We have shown that biological membranes have low, selective permeability
retaining proteins and allowing passage of inorganic substances
(electrolytes, water) and of organic substances with small molecule.
The proteins with amfoter character but with a
dominant negative charge can form compounds with cations of the R – Na+
type. These types of compounds prevent the uniform distribution of
electrolytes on both sides of the semipermeable membrane in accordance with
the laws of osmosis. This action of the resulting compound is proportional to
its concentration and may even stop the passage of electrolyte when R
concentration is very high. This special behavior of osmosis, induced by
non-diffusible proteins is identified as "Donnan’s equilibrium",
expressing the unequal distribution of ions on both sides of the membrane.
This balance explains the quantitative differences of electrolytes between
blood and CSF, between red blood cells and plasma, etc. The decrease in protein content alters
the allocation of water and electrolytes. The unequal distribution of
undiffusible ions creates a difference in the membrane potential, and the
unequal distribution of electrolytes explains the existence of membrane
potential both at rest (resting potential) and in action (action potential.
|
|
 i
|
|
|

|
Sugeraţi
|
|
|
|
|
|
|
2.3.3. The stability of plasma solution.
The solubility of macromolecular compounds and consequently of plasma compounds
is preserved with no addition of stabilizer agents as required by colloids. The
stability of the plasma solution is provided by water and by two other
categories of factors: the chemical and the physical factors that condition its
physiological properties
2.3.3.1. Chemical factors of stabilization.
The solubility of proteins depends on
their Nature and chemical structure, the solution pH, the degree of ionization,
the ionic composition of the environment (salt concentration and Nature of
salts), the dielectric constant of the environment, the ionic composition of
the environment, including the type of side groups of protein. The role of the
solvent: water is bound by polar interactions to the ionized groups and by the
hydrogen bond to the peptidic bond. Water binding is minimal at the izoelectric
pH of proteins.
Electrolytes in low concentration favor
protein solubilisation and stabilize the solution. If the electric charges on
the protein molecules are neutralized by the addition of salts, proteins
precipitate by salefier (178). Electrolytes in high concentration destroy the
water interaction with the protein molecule and separate them. Non-electrolytes
may also dehydrate macromolecules through interaction with water and decrease
the solubility of proteins through a process of pseudo-hyper concentration.
The ability of electrolytes to influence water
dissolving power follows Hofmeister's liotropic series. (52):
Li> Na>
K> Rb> Cs
The solubility of the proteins
increases in solutions with high a dielectric constant (52) and in the presence
of dipole ions.
An important role is also played by the attraction and
repulsion forces existing on the surface of molecules in solution. In the two
segments, arterial and venous, there occur composition changes of water,
electrolytes, various other molecules that can cross the capillary wall as well
as a result of loading with catabolites and other products of local secretion.
Also at this level, there occur variations in the concentration of
macromolecules retained by the capillary wall, as, for example, the increase by
20% of the concentration of protein in
the blood during the formation of primary glomerular ultra filtrate. H-ions
concentration increases in venous plasma.
2.3.3.2. Physical factors of plasma stability.
There are many physical conditions of stability. Among them one could list
Brownian motion, continuous flow of blood, rheology factors depending on the
type of blood flow in vessels, etc. The flow of a liquid through a closed
system of straight, cylindrical tubes is laminar. The liquid is moving in
parallel, immiscible layers alongside the vessel wall. Otherwise, the layers
are mixed, form whirling areas where plasma is shaken. In these areas the lumen
of the vessels is altered naturally (the valvular cardiovascular blood
pressure, fast-growing areas of vascular bed, and under conditions of temporary
exertion of some organs in the precapllary region), or in pathological
conditions. An important physical factor is represented by the large specific
surface of the solvite substances (relative to the surface, the particle size
with an average of 3.5 mμ of a gram of substance). The surface grows even
larger as a result of hydration (178).
2.3.4. Plasma functions (78, 136) are listed only:
(A) - maintain the physical and chemical properties of blood.
(A1) - maintain colloid osmotic pressure
. (A2) - monitor the exchange of electrolytes between plasma and interstice.
(A3) - maintain blood viscosity.
(A4) - maintain acido-basic balance and the pH of blood.
(A5) - maintain blood volume.
B) - the body's defense mechanism and the immune response.
(C) – own enzyme function: coagulation, fibrinolysis, etc..
(D) - ensure common fund of proteins and amino acids and ensure balance
with tissue proteins.
(E) - transport function for amino acids, polypeptides, fats,
carbohydrates, vitamins, minerals, electrolytes, hormones, bilirubin,
metabolites, gas, etc.
(F) regulatory function:
(F1) - maintain the intra- and extra-vascular hydro - electrolytic
equilibrium and general homeostasis.
(F2) – ensure humoral unity of the body alongside the nervous path.
2.3.5. Plasma proteins.
2.3.5.1. General information
Proteins are chemical compounds
with high molecular complexity and variation (1o
-10
types of proteins in the living world) and special
significance (69). The fundamental elements of proteins are amino acids, the
peptidic bond, polypeptide chains and functional groups alongside the
polypeptide chain. The polypeptide chain is composed of a skeleton consisting
mainly of carbon chains of amino acids associated through peptidic bonds.
The chain variation is given by radicals "R" of amino acids. The
uni-dimension chains are flexible as the covalent bond allows free rotation of
the carbon atoms. This allows a spontaneous conversion to a three-dimensional
structure typical of a specific sequence of amino acids. Spatial organization
of constituents of proteins is achieved by noncovalent interactions, which also
involve groups in the peptidic bond and other groups in the side chain of the catena:
Coulomb interaction, Van der Waals'force, London's dispersion forces, hydrogen
bonds, and some configuration of the polymers The hydrogen bonds are
established following privileged directions imposed by the strongest direction
to the colinearity of the three atoms involved in the link. Most proteins are
spontaneously refolding after their prior unfolding (denaturizing), which shows
that all the molecular information is contained in the sequence of amino acids.
The folding of the polypeptide chain is
conditioned by the amino acid composition and the distribution of polar and
unpolar groups (Table No. 11). They control the folding of protein molecules
(160124,15,16,17). Hydrophobic sites are accommodated
inside of the molecule thus avoiding contact with water and creating globulin
structures. Hydrophilic polar side chains are distributed on the external
surface where they can interact with water and other polar molecules. The
hydrogen bond is important in keeping together the various segments of the folding
molecule.
The additional covalent bonds between the chains and
disulfuric bridges contribute to the stabilization of the three-dimensional
structure of the extra cellular proteins. Molecular specificity results from
the interactions between their various structural elements and the molecules in
their environment. They are based, in addition to covalent bonds, on hydrogen
bonds, phosphate bonds, saline bonds, etc.The distribution of various atoms on
the surface of the molecule, makes each protein unique and able to interact
with specific molecular and other surfaces and with some smaller molecules.
Each protein has different segments that are repeated
in the macromolecule. The polypeptides, in the solution, take a unique spatial
arrangement as a result of noncovalent interactions in the protein so that its
energy could be minimal. Noncovalent bonds are established very quickly with no
catalysts and they are effective by aggregation. Inside the protein molecule
there is a dielectric environment which favors interactions, is associating to
reduce contact with water, forming a large number of contacts unpolar.
2.3.5.2. The structure of the protein.
Each species is unique protein in the primary structure and conformation, and
the radicals compete to achieve the structure of tertiary and quaternary field.
The complexity of proteins required recognition of several steps of
organization structure (53,160): (1) primary, (2) secondary (3) tertiary, (4)
and quaternary.
2.3.5.2.1 The primary structure.
The primary structure defines the number and sequence of the amino acids
residue, the totality of peptide bonds in the polypeptide chain. It expresses
the message described in DNA. The primary genetic structure is unique and
compatible with a specific function of the protein. If an amino acid is
replaced with another one in the same group (conservative substitution) or from
another group (unconservative substitution), homologous proteins appear.
Molecular polymorphism defines the existence of multiple molecular structures
for the same function in the same species.
Inter-atom
measurements of distances and angular values connecting the amino acids in the
polypeptide chain established the following primary characteristic of
structure:
- The peptide bond has the character of double bond by the conjunction of
orbitals p.
- Free rotation around the peptide bond and carbon can take an equal footing in
the plane of the bonds.
- Natural peptides in the configuration trans are more stable than in the
configuration "cis"
- the group = NH forms hydrogen bonds with the group C-O from other
peptide bond to form NH ... O = CH.
2.3.5.2.2. The secondary structure.
A polypeptide chain can exist in two configurations:
and
. The helicoid-
structure results from spinning of the polypeptide chain to
the peptide bonds so that the groups O=C and =N-H are adjacent and can form
hydrogen bridges and helicoid repetitive units. A group =N-H forms hydrogen
bonds with group O=C of the fourth residue of amino acid in the same chain of
linear sequence. The peculiarities of the alpha-helix structure are determined
by the bonds angles, interatomic distance, co linearity of the hydrogen bonds,
series optical levogyrous. These features are: (1) With every amino acid
residue the length increases by 1.47 Ǻ. (2) the helix pitch is 5.21 Ǻ
and contains six amino acid residues. (3) diameter of
the helical cylinder where the carbon atoms are located is 10.1 Ǻ. (4) the
sense of rotation is from left to right. (5) radicals
"R" of all amino acids are oriented towards the outside of the helix,
(6) all =N- and =CO groups form hydrogen bonds. (7) polypeptide
chain has the shape of a stick with a diameter of 10.1 Ǻ for 300 amino
acid residues.
The residue of proline prevents the
- spinning, and the chain is bent at an angle of 130
. The residue glycocol (no side chain) confers fexibility
interrupts the structure
, and changes slightly the chain direction. Other amino
acids: valine, isoleucine, treonine induce steric disturbances. Serine forms
hydrogen bonds at alcoholic group and prevents the helix stability. Cysteine
creates disulphur briges and a rigid polypeptide chain, hindering formation of
the helix. The
- structure has the shape of a folded sheet. In this
structure the maximum of hydrogen bonds is achieved between CO and NH. The
hydrogen bonds are intercatenary and polypeptides are arranged in the shape of
sheets. The most stable configuration is the structure
with antiparallel chains (chains are directed from the
"N" terminal to the C terminal and the other vice versa).
Radicals "R" are arranged on alternating
sides of the polypeptide chain. Between sheets there are hydrogen bonds
connecting the "R" groups. Distances between the sheets are 5.7 and
3.5 Ǻ
alternatively. The structures
are frequent in globular proteins, especially in immunoglobulins.
A simple
- structure is formed of a polypeptide chain bent on itself, forming two antiparallel segments identified as the
-tower.
2.3.5.2.3. Tertiary structure.
This includes the secondary structures as spatial
units: domain and motifs. The packaging is noncovalent and by carbon
interactions between the radicals "R" at C
of the structure
,
or unorganized. The bonds are determined as follows: (1)
hydrogen bonds between the OH groups of the amino acid residue tironyl,
tirosyl, with amide groups of glutamyl and asparaginyl (2) ionic bonds between
radicals with negative charge of lisinyl, arginyl, histidyl with and without
polar radical charge (3) hydrophilic interaction between the residue unpolar
amino acids valine, leucine, isoleucine, alanine, phenylalanine (4) links
between the cysteine residue distant covalent regions of the molecule.
A polypeptide chain adopts the configurations
and
until it satisfies all
the affinity of radicals "R" under steric conditions influences when
the final conformation results, the best possible compromise of stable energy
and space. Therefore, the decisive factor to obtain the tertiary conformation
given is again its genetically induced primary structure whose primary
spontaneous structure depends on the chemical composition. The plasma globular
proteins have on polar groups on their surface. The unpolar radicals are
included within the protein molecule.
The organization of the "domains" is a
structural intermediate form between the secondary and tertiary structures.
Because of this, it is identified as "secondary superstructure" or
"superstructure domain." Domain "is a continuous piece of the
primary structure of polypeptide chain, packed up as a functional entity with
its own secondary and tertiary structure. The "domains" are part of
the less organized part of the chain, allowing flexible dynamic segments of
their field. "Domains" are packagings of
-helix and
- sheet, forming globular compact units. It contains a number
of 50 - 350 amino acid residues. Protein may be constituted in a domain or more
connected with each other through long chains of open polypeptides. Through
these connections large molecules are formed, identified as “protein ensembles”
or “protein complexes” in which the subunits are linked with a large number of
noncovalent bonds. In the extra cellular environment they are often stabilized
by disulphur bridges. So a "domain" of protein appears as a basic
core of a protein which is composed mainly of a
- sheet,
-helix or both. These structures allow long hydrogen bonds
that stabilize the inside of the molecule where water has no access to form
hydrogen bonds with the oxygen or polar carbon with the hydrogen of peptide
bond. Proteins can be formed through recombination of pre-existing polypeptides
"domains" with supramolecular structures of complex type enzyme,
protein filaments, membranes, etc. Domains are the underlying structure of
proteins diversification. They are the basis of "analogous" proteins
(different proteins with similar functions) and of "homologous"
proteins (similar proteins with different functions).
2.3.5.2.4. Quaternary structure.
This represents associations of protomers (polypeptide chains with their own
structures: primary, secondary and tertiary) identified as
"oligomers". The specific function of an oligomer protein is
manifested only at the level of quaternary structure. The separated protomers
are inactive.
The assembling of the protomers to oligomers is
accomplished only when the contact areas are complementary and have a large number
of atoms, and the bonds are close to the level where the Van der Waals are
active. The protomers of homologue proteins of different species do not
associate although they contain the information to be decoded. The interaction
capacity explains the formation of supramolecular structures. Perturbation of a
protomer annihilates the oligomer function.
2.3.5.3. Electric charge of proteins.
The presence of amino and carboxylic groups of amino acid residues gives
proteins their amphoteric properties. The solution can be split as acids or
bases as having both negative and positive charges. Sign and size of the
proteins charge are not constant but depend on the composition of the
environment and in particular on the pH of the solution. The behavior of protein
macromolecules [Michaelis] in water can follow two schemes:
(a) in an alkaline environment H+ is
released, so it acts as an acid (see also acid-basic equilibrium):
[R-(NH) (COOH] + Na + OH [R-(NH) (COO] Na + HO
(b) in an acid environment(HCl) with an excess of H+ which are
additional, the amine group as a base is loaded positively:
[R-(NH) (COOH] + H + Cl -------- [R-(NH) (COOH1 + Cl
In both cases a reaction of neutralization is
described, with formation of salts: Na-protein ate, in the first case (178, 68),
protein clorhydrate (macrocation) in the second case.
This is also seen during the electrophoresis of the protein solutions. It
suggests the representation of proteins as ion amphoters or amfions [R. (NH)
(COO)] --------- [R - (NH) (COOH), the two forms being in equilibrium.In a
neutral state, the linear amino acids are in the form of amfions. The behavior
of amfions can be written like this:
R (NH +) (COO) + OH------------
[R-(NH) (COO] + HO in an alkaline environment. In an acid environment, H+
in excess H is in added to the NH grouping which is loaded positively.
R-(NH2)
(COOH) + H --------- [R - (NH )(COOH)]
The isoelectric point represents the state of electric potential
"zero". In solutions of proteins it depends directly on the total
environment pH and indirectly on the concentration of other ions in solution.
Note that the isoelectric pH of the protein molecule is not constant and
depends on the Nature of the amino acid composition of the protein molecule.
Also, even when number of NH = COOH, the isoelectric pH is slightly on the
acid side. Electrochemically, proteins are macromolecular ampholytes. The
most important contribution to the acidic and basic charges belongs to the
residues of glutamyl, aspartyl, lizyl, arginyl and histidyl. The contribution
of terminal "C" and "N" is reduced as the length
polypeptide chain increases. These electrical charges can be modulated in
solution to be separated electrophoretically.
|
|

I2.3.5.4. Protein denaturizing
The denaturizing of the protein is a structural modification where molecular
weight and covalent bonds are preserved. Denaturizing is of interest to
noncovalent bonds specific to the Native conformation. Denaturizing is induced
by physical agents (temperature above 60 C, with high frequency radiation as ultraviolet rays,
X-rays, strong shaking, hydrostatic pressure, etc.), chemicals (acids, bases,
organic solvents, detergents, etc.), and biological stimuli acting in vivo.
By denaturizing, the solubility of the protein decreases to the level of
precipitation, it also lowers the colloid-osmotic pressure, their viscosity,
optical activity, there is increased sensitivity to the action of enzymes
some of their biological functions are inactivated (enzyme, hormone
replacement therapy). The mechanism of denaturizing depends on the Nature of
the agent. After removal of the agent (in vitro), the protein returns to its
original state. During stimulation of excitable structures in vivo and during
transmission of information message, there occur changes in the status of
physical-chemical properties of cellular protein, identified as "Tran
conformational changes" (16, 17) which are reversible after a while by
metabolic and genetic mechanisms.
Such changes occur during the reflex stimulation of
the cerebral cortex in rats (14, 16, and 17) as a decrease of protein
solubility in the stimulated area, moving the buffer capacity of proteins
towards the alkaline side, thus lowering the capacity of ionization of the
carboxylic groups of protein from the stimulated cortex. The same changes
occur in old, non-stimulated rats, compared with adult rats. Therefore, the
processes of aging are accompanied by changes in the brain proteins and
soluble endocellular potassium is depleted. Restoration of the structure of
proteins takes place with a very small consumption of energy (15, 16, 17).
|
|
|
2.3.5.5.
Methods of dosing proteins.
In order to individualize blood proteins molecular parameters are used: size,
molecular weight, electric charge, chemical bonds, the complexity of
structural complexes with other substances, conformation, the Nature and
number of reactive groups, behavior in different environments, ion
concentrations at various temperatures, the identification of their function
as transporters, the biological properties as antigenicity and their immune
characteristics. About 100 protein
fractions were identified in blood. Below is a brief review of some methods
used in clinical practice and research.
Methods of quantification: Kjeldal methods (determine total nitrogen in
protein), colorimetric methods (burette reaction), spectrophotometric methods
based on protein interaction with light (especially ultraviolet),
spectrophotofluorimetric methods based on stimulated emission of light by
proteins that contain aromatic amino acids, refractometric methods.
2.3.5.6
Methods of separation
2.3.5.6.1. Precipitation with neutral salts of different concentrations:
NaCl, PO K, Na SO (SO (NH), by precipitation to isoelectric point, or ethanol
in the cold, etc...
2.3.5.6.2 Methods of electrophoretic separation and characterization.
These methods are based on the property of proteins to become selectively
ionized in various conditions of pH, and thus to migrate to a variable
electric field. This method can be combined with other procedures thus
enhancing its capacity of providing information. It can use various supports.
|

|
|
|
|
|
|
|
|
Electrophoresis in a liquid phase
(Moving boundary electrophoresis Tselios). It was used by us as well for research activity. It requires a
Tisselius apparatus. It is using 1 -2 ml of blood serum that is diluted with 2
ml.buffer Michaelis-Wideman, pH = 7, 4, ionic strength = 0.118. First, it is
dialyzed in buffer at 40 C for three hours. Dialysis continues for a
second time for another hour after changing the buffer. Direct current of 150
volts is used.
Two photographic exposures of the two branches of the
device are performed, upward and downward, after 45 min. and 75 min. migration.
One calculates the mobility (fig. 1) of the fractions obtained, with the
equation:

= V / E = ds / dt where:

= Electrophoretic mobility of the "i" fraction.
S
= space covered by the fraction "i" in the 30 min.
interval
E = electric field strength in the cell (30 V / cm).

, represents the average mobility in the two branches
Figure 1.Electrophoretic mobility (
=cm
/V)of three plasma protein fractions
of normal children and children with behavioral constitutioNal disorders and
transport of Na in the ionic state. (230)
Figure 2.Schematic representation of the serum electrophoresis obtained by four
methods: (a) Tisselius electrophoresis, (b) electrophoresis on paper (c) gel
electrophoresis in starch (d) immunoelectrophoresis (160).
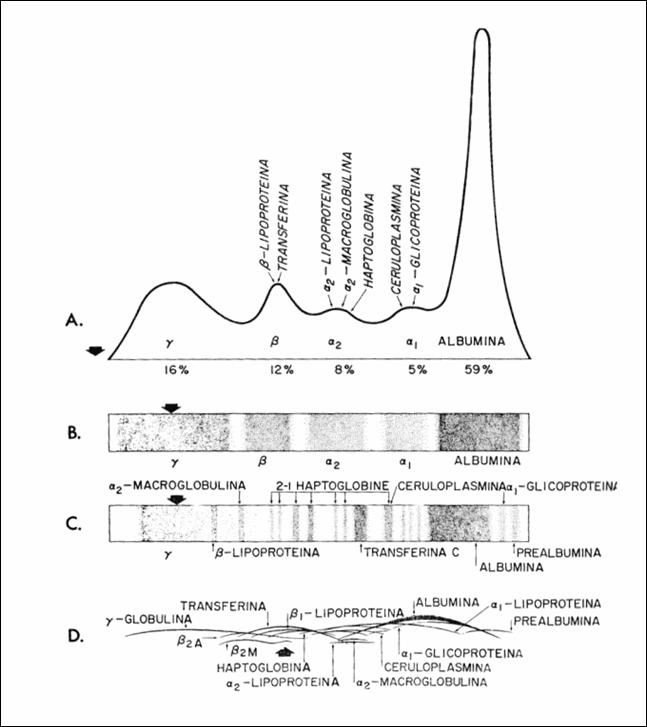
In Figure 2, vertical arrows indicate the point of departure
in gel electrophoresis in starch but moving in the field of departure in each
case. Globulin remains the starting point in the gel electrophoresis of starch
but is shifting in the domain
, in other metods.Other methods use starch or agar gel as
support, with good results in imunoelectrophoresis.Imunoelectrophoresis allows
identification of the areas having globulin immunochemical specificity.
2.3.5.6.3 Centrifugal analysis
of serum.
The method takes into account the molecular weight and environment density. The
method allows a good separation, if centrifugation is performed in solution
with gradient of concentration. It is especially useful to separate
lipoproteins.
2.3.5.6.4 Chromatographic
Methods
These methods are based on the adsorption properties of hydrophilic ion
exchangers. Given the ionic properties of proteins, exchange and buffers for
adsorption and elution are almost invariably used. Adsorption chromatography
involves substance deposition on solid surfaces, followed by differential
elution. Between the solids (bed selected) and proteins various interactions
are established: ion, hydrophobic, hydrogen bonds, etc. The most important are
the exchanger-ion interactions. Elution is performed by washing or by lowering
the pH, which alters the number or sign of protein (or adsorbent) charge, or by
increasing the concentration of salts which decrease the strength of bonds
between proteins and adsorbent. In other systems hydrogen bonds are generated
between the carboxylic group of proteins and the non-ionized carboxylic groups
on the ion exchanger. Their affinity is controlled by adjusting the pH to
adjust the number of non-ionized carboxyl on protein and resin. Resins
(Amberlite XE) have very high density of non-ionized carboxylic groups,
compensating to some extent the fact that only groups on the surface are
available to bind the proteins. Hydrophobic bonds appear less frequently, but
they strengthen the bonds of the primary adsorbents, ionic or hydrogen bonds.
If many interactions are formed between the adsorbent and the protein, the
latter will not move from where it is connected as long as conditions remain
unchanged, and the likelihood of these links to simultaneously dissociate is
small. Therefore affinity of the protein for the adsorbent will gradually
decrease to zero and therefore one obtains a range of protein unbinding. For
several proteins, there appear several equilibrium constants. The affinity of a
protein for an ion exchange is expressed by the number of links between
proteins and adsorbent and these are useful for separating large
molecules.Amberlite XE-64 is a cationic exchanger that allows the exchange with
negative proteins. In amphoteric hydrophilic gels the binding forces are weak.
So the number of charges on the molecule and their space distribution are
criteria of differentiation of proteins.
Different proteins have different ways to change the
variations of pH. The adsorbents can be very different, and eluents infinitely
increase the technical possibilities. Ion exchange resins (Dowex 1 and Dowex
50) have ion charges on the surface of the calcium phosphate gel type. The
cellulose ion exchange has ionizing groups with affinity for many proteins. The
covalent bonds allow cellulose stability ionizing groups. An example of the
group is DEAE cellulose and carboxymethyl cellulose, an ion exchanger for basic
proteins. Phosphorilate cellulose (P cellulose) and sulfoethyl cellulose
(SE-cellulose) are cationic exchangers with stronger acidic groups that retain
the negative charges to very low pH. Other derivatives are guanetidil cellulose
(G-cellulose) for very high pH, triethylaminocellulose anionic exchanger, sefadex is an ion exchanger with cross-links.
Chromatography with molecular sieve or gel filtration uses sefadex, gel of
granulated polyacrilamid, agar and agarose. So this is a way of sifting
molecules by size, along with electrochemical separation. The more sensitive
methods used for detecting and dosing are the radioimmunologic (RIA) and the
immunoenzymologic ones (ELISA).
2.3.5.7. Classification of plasma proteins
At the basis of plasma proteins classification (62)
are the results of research with all the methods applied, discussed above.
There are holoproteins consisting of chains of amino acids only, such as
albumin, and heteroproteins which have a prostetic fragment: carbohydrates,
lipids, metals in glycoproteins, lipoproteins, metalproteins
respectively.
2.3.5.7.1 Electrophoresis classification.
Migration in the electric field is in Veronal buffer pH = 8.6. Other buffers
can also be used, but the basic condition is that the pH should be as far away
as possible from the piezoelectric point. Albumins also migrate in a pH = 7.4,
equal to the normal pH of the blood.
Table 5. The main plasma proteins isolated by
electrophoresis (175)
Electrophoresis fraction
|
values %
|
gr/dl
|
Prealbumin
|
|
|
Albumin
|
5, 2 – 5, 9
|
3, 5 – 5, 5
|
 -globulins
|
3, 5
|
0, 25-0, 35
|
 -globulins
|
8, 0 – 1o, o
|
0, 50-0, 75
|
- globulins
|
12, 0 - 14, 0
|
0, 80-1, 05
|
- globulins
|
16, 0 - 20, 0
|
1, 10-1, 50
|
Another classification is based on the rate of sedimentation in the centrifugal
field in liquid medium with or without gradient density. The use of a large
number of physico-chemical characteristics of the protein fractions allows the
identification of fibrillary proteins that adhere to the vessel wall.
2.3.5.7.2. Other classifications
A classification highlights their immunological value.
Another class is active in the processes of haemostasis. Other proteins form
the signalers group and yet another, the group of receptors.
2.3.5.7.3. Classification as carriers
Many proteins are transporters, ensuring the takeover
of ligands: hormones, amino acids, chemical mediators, electrolytes, Na. (92,
160). From the molecular parameters, (electrophoretic mobility, izoelectric
point, molecular weight, the sedimentation constant, the diffusion constant,
partial specific volume, viscosity, friction rate), one established molecular
polymorphism ranging from the fibrillar elongated form, insoluble in water to
hydrophilic, globular forms. Molecular weight of plasma proteins is also a very
heterogeneous parameter; their values are between 44,000 and 1,000,000. The
determination of molecular weight of plasma protein is difficult because they
easily form aggregates, dimers with different weights, or they dissociate
during their storage. When injected intravenously, small forms are easily
removed in the urine. If they have more acidic groups, elimination is hindered.
Table 6 the percentage content of prostetic groups in
certain plasma proteins (132)
Protein
|
% sugars
|
% lipids
|
physiological role
|
Prealbumin
|
1, 3
|
0, 2
|
tyrosine fixing
|
Albumin
|
0, 0
|
0, 0
|
Transportation substances
|
Orosomucoid
|
41, 4
|
0, 0
|
|
 macroglobulin
|
8, 5
|
0, 0
|
„Transport” ?
|
haptoglobin
|
18, 6
|
0, 0
|
Substance transport
|
Ceruloplasmin (content Cu =0, 84%)
|
7, 8
|
0, 0
|
Oxidase
|
Transferrin
|
5, 9
|
0, 0
|
Iron transport
|
 lipoprotein
|
1, 5
|
55, 0
|
Lipids transport
|
 lipoprotein
|
1, 7
|
90, 0
|
Lipids transport
|
 lipoprotein
|
1, 8
|
80, 0
|
Lipids transport
|
globulin
|
3, 1
|
0, 0
|
Immunity
|
One notes the better expressed transporter role of
proteins with sugars as a dominant group, the transport of lipids belonging
more especially to lipoproteins.
[The remainder of Chapter Two will be
featured in the upcoming September-October issue of this Journal.]
Professor Marcel Uluitu, M.D. Ph.D. began his
scientific activity in Physiology in 1953 at the Physiology
School (Medical
School) Cluj-Napoca,
in Romania.
He continued his scientific activity in Physiology in Bucharest at the
Institute of Physiology and Pathophysiology Daniel Danielopolu until 2004, at
which time he retired as Director of the Institute; he had held this position
since 1990. His research work includes studies of the central nervous system
physiology relating to the transmission of information in the nervous centers;
cerebral excitability, using methods, in their evolution, from the
determination on reactive isolated organ, to the most complex physical and
chemical methods, in the present. In his research of chemical mediation he
studied acetylcholine, serotonin, and their connection with the cerebral
metabolism.
Professor Uluitu has also investigated
cerebral tissue excitability, studying the structure modification of the
protein macromolecules, and the physiological and pathopysiological processes
in which are involved Sodium and Lithium. He implemented an original method for
physical and chemical processes which involve the chemic active sodium, in
normal processes and in the cerebral excitability dysfunctions, in human and in
experimental model (animal). These results of this work gave him the chance to
outline the chapter herein relating to the physiology of substances transport
in the blood. This is based on the physical and chemical interaction between
blood components.
His papers are included in the
collections of the U.S. National Library of Medicine and the U.S. National
Institute of Health. He is a member of the Romanian Academy
of Medical Sciences.
Dr. Diana Popa (Uluitu) is a
researcher in the Department of Microbiolgy, Immunology and Molecular Genetics
at the University of Kentucky in Lexington,
Kentucky, USA.
She attended Medical School at Vasile
Goldis University
in Arad, Romania,
and graduated in 1998; during summer breaks at medical school she volunteered
at the Institute
of Physiology D. Danielopopu
in the phyisiology and microbiology laboratories as well as in the clinic.
After completing medical school and a one year internship, she worked as a
Research Assistant at the Danielopopu Insitute for three years before coming to
the University of
Kentucky. In addition to
her medical thesis, which focused on the actions of Lithium on the central
nervous system, since coming to the United States she has published
numerous additional papers relating to Lithium and other substances.